- 1School of Medicine, Jiangsu University, Zhenjiang, China
- 2Department of laboratory Medicine, School of Medicine, Jiangsu University, Zhenjiang, China
Gastric cancer (GC) is one of the most pernicious gastrointestinal tumors with extraordinarily high incidence and mortality. Ubiquitination modification of cellular signaling proteins has been shown to play important roles in GC tumorigenesis, progression, and prognosis. The E3 ubiquitin ligase is the crucial enzyme in the ubiquitination reaction and determines the specificity of ubiquitination substrates, and thus, the cellular effects. The HECT E3 ligases are the second largest E3 ubiquitin ligase family characterized by containing a HECT domain that has E3 ubiquitin ligase activity. The HECT E3 ubiquitin ligases have been found to engage in GC progression. However, whether HECT E3 ligases function as tumor promoters or tumor suppressors in GC remains controversial. In this review, we will focus on recent discoveries about the role of the HECT E3 ubiquitin ligases, especially members of the NEDD4 and other HECT E3 ligase subfamilies, in GC.
Introduction
Ubiquitin is an evolutionarily conserved 76-amino acid polypeptide, which serves as a modifier molecule that covalently binds to target proteins for degradation or non-degradative signaling (1). The process attaches ubiquitin to specific substrate proteins, which is called ubiquitination, and is one of the most important protein post-translational modifications. Ubiquitination has been shown to regulate and maintain basic biological functions, including cell cycle, cell differentiation, cell localization, cell survival, apoptosis, signal transduction, and the inflammatory response (2-4). Ubiquitination, including activating, conjugating, and ligating, is a highly orchestrated enzymatic cascade involved in the multistep processes catalyzed by activating enzymes (E1), conjugating enzymes (E2), and ubiquitin ligases (E3) (5). In the human genome, two E1s, 38-40 E2s, and more than 600 E3 ubiquitin ligases have been identified (6,7). E3 ubiquitin ligases are remarkable enzymes, which directly medicate the interaction between ubiquitin and substrates, and mainly determine the substrate specificity and versatility for ubiquitination modification (8,9). In mammalian cells, E3 ligases are usually the prominent focal point of cell regulation, which makes them the most attractive targets for disease treatment and intervention (10,11). HECT E3 ligase activity and substrate specificity can be regulated by a wide variety of mechanisms, such as different post-translational modifications or protein–protein interactions (12). There are several approaches for targeting the HECT E3s, however specific inhibitors of the HECT E3s have still not been exploited.
Gastric cancer (GC) is an extremely aggressive tumor and is estimated to be a major cause of cancer-related death worldwide (13,14). The pathogenetic mechanisms of gastric cancer are complex and heterogeneous, involving environmental factors, gene mutations, copy number variants, and epigenetic changes (15,16). Recently, the HECT E3 ligases have received considerable attention due to their multiple roles in the occurrence and development of GC. Due to the scarcity of studies concentrating on the potential contributions of HERC subfamily E3 ligases to GC, in this review we focus on the roles of NEDD4 subfamily and other HECT E3 ligases in GC development (Table 1).
The HECT E3 ubiquitin ligases
Based on their structures and catalytic mechanism, E3 ubiquitin ligases can be categorized into three groups: HECT (Homologous to E6-associated protein C-terminus) E3s (44-46), RING (really interesting new gene) E3s (47,48), and RBR (RING-between-RING) E3s (49,50). RING E3s, which make up 95% of E3 ligases, act as scaffold proteins, performing a single-step ubiquitin transfer from the ubiquitin-charging E2 enzyme to the substrates (45). Unlike RING E3s, HECT and RBR E3 ligases contain catalytic cysteine residue, forming an intermediate thioester bond with ubiquitin before the transfer of ubiquitin to lysine residue on the substrates (51).
HECT E3 ligases have a total of 28 members, typically with a conserved ∼40 kDa HECT domain, which can be further divided into three subgroups on the basis of their domain architecture in the N-terminus:NEDD4 subfamily with C2 and WW domains, HERC subfamily with RLD domains, and “Other” HECT E3 subfamily which contains neither C2 and WW domains nor RLD domains (12,52) (Figure 1).
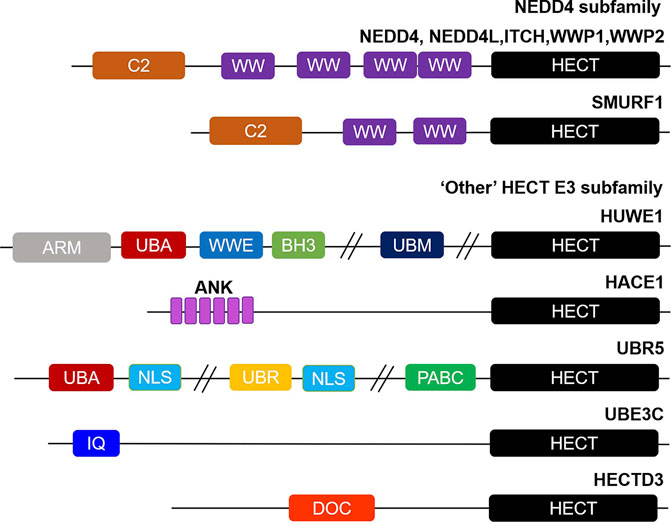
FIGURE 1. Overview of structural domain organization of HECT E3 family members. HECT E3 ubiquitin ligases are grouped into three subfamilies: NEDD4, HERC, and “Other” HECT E3 subfamily on the basis of their N-terminal structural properties to the HECT domain. The NEDD4 subfamily is characterized by C2 and WW domains, and “Other” HECT E3 subfamily carries a myriad of structural domains in their N-terminal region. The domain abbreviations used are as follows: C2, Calcium-dependent lipid binding domain; WW, WW domain; HECT, homologous to E6AP C-terminus; ARM, armadillo repeat-containing domain; UBA, ubiquitin-associated domain; WWE, WWE domain; BH3, Bcl-2 homology 3 domain; UBM, ubiquitin-binding motif; ANK, Ankyrin repeat-containing domain; NLS, nuclear localization sequence; UBR, ubiquitin-recognin box; PABC//MLLE, polyadenylate-binding protein C-terminal domain; IQ, IQ motif/EF-hand binding site; DOC, APC10/DOC domain.
The NEDD4 subfamily is the most famous and has been studied most in-depth of the HECT E3 ligases, consisting of 9 proteins in humans: NEDD4 (NEDD4-1), NEDD4L (NEDD4-2), ITCH (AIP4), WWP1 (AIP5), WWP2 (AIP2), SMURF1, SMURF2, NEDL1 (HECW1) and NEDL2 (HECW2) (53). Structurally, NEDD4 subfamily proteins contain an N-terminal C2 domain, two to four WW domains in the central region, and a C-terminal HECT domain (Figure 1). The C2 domain is defined as a Ca2+ and is a phospholipid binder involved in protein-protein interactions, regulating the activity of the HECT domain, as well as membrane binding (54-58). The WW domains have two conserved tryptophan residues, which are thought to be mainly responsible for protein-protein interactions via interacting with proline rich PPXY motifs and phosphorylated Ser/Thr residues in substrates (59-61). Whereas the HECT domain exhibits catalytic E3 activity and catalyzes the transfer of ubiquitin to substrates. The HERC subfamily can be divided into large and small HERCs. The large HERCs include HERC1 and HERC2 that contain more than one RLD domain and additional predicted domains. Whereas the four small HERCs, named HERC3∼6, only have a single RLD domain. RLD domains can interact with chromatin through histones H2A and H2B, in addition to functioning as a guanine nucleotide exchange factor (GEF) to regulate the small GTPase Ran (62,63). Finally, the 13 members of other HECT E3s carry a myriad of structural domains in their N-terminal region that are responsible for binding and recruiting substrates (64) (Figure 1). EA6P, HUWE1, UBR5 (EDD), TRIP12 (ULF), and HACE1 are the best studied of other HECT E3 ligases. It is worth emphasizing that with the development of sequencing technology, it may be possible to further subdivide the ‘other’ HECT E3s into subfamilies according to their genetic evolutionary histories in the near future (65,66).
NEDD4 subfamily
NEDD4
Neuronal precursor cell-expressed developmentally downregulated 4 (NEDD4, also known as NEDD4-1) is the ancestral and prototypic member of the NEDD4 family, and has been unveiled as a versatile E3 ligase with an extensive range of target substrates. NEDD4 is thought to be implicated in various human diseases, from cardiovascular disease (Liddle’s syndrome) to cancers (61,67). Interestingly, the relationship of NEDD4 to GC appears to be rather complicated. One study showed that overexpression of NEDD4 was detected in 75% GC tissues (17). Surprisingly, there is no association between NEDD4 levels and invasion, metastasis, or stage. However, another study found that NEDD4 is upregulated in advanced GC tissues and associated with metastasis (18). In accordance with the study, we found that gastric cardia adenocarcinoma patients have a high expression of NEDD4, which was associated with metastasis occurrence and negative prognosis of patients (19). Unexpectedly, Yang et al. observed no upregulation of NEDD4 and no correlation with PTEN expression in GC tumor samples (20). The discrepancy may be due to the differences in gastric tumor types, stages, or sample numbers. Therefore, it is worth exploring the role of NEDD4 in GC further.
NEDD4L
Neural precursor cell-expressed developmentally downregulated 4-like (NEDD4L, also known as NEDD4-2) is highly homologous to NEDD4 and is thought to have originated later in evolution, as it has a different substrate repertoire from NEDD4 (68). To date, NEDD4L has been implicated in carcinogenesis and tumor progression by regulation of multiple central pathways, including TGF-β, PI3K-AKT, Wnt, and EGFR signaling pathways (69-72). GC patients were observed to express low levels of NEDD4L with an aggressive clinical course and poor clinical outcomes (21). Additionally, NEDD4L is an independent predictor for GC metastasis and survival. At the same time, the expression of NEDD4L protein in GC tissues is negatively correlated with HIF-1α, suggesting that NEDD4L may, together with HIF-1α, contribute to metastasis of GC (22), but the underlying molecular mechanism remains unclear.
ITCH
ITCH, also known as AIP4, was initially identified as an important immune system regulator (73). Emerging studies have revealed a critical connection between ITCH and tumorigenesis, and GC as well. ITCH is reported to ubiquitinate and degrade SMAD7 (74,75). Gen et al. have demonstrated ITCH activity is restricted via its interaction with ASSP2 that exerts an inhibitory competitive action on the recruitment of SMAD7, a key gene in TGF-β1-SMAD2/3 signaling, which in turn inactivates TGF-β1-SMAD2/3 signaling to inhibit invasion and TGF-β1-induced EMT in diffuse-type GC (23). However, a recent study found Cir-ITCH may increase ITCH expression by sponging miR-17 (24). Moreover, Cir-ITCH significantly decreases the protein level of β-catenin and p-Dvl2 and two Wnt target genes c-Myc and cyclin D1, thus attenuating the cell proliferation, migration, and invasion in GC (24). Clinically, GC patients expressing low levels of Cir-ITCH and ITCH with metastasis have shorter overall survival times (24,25). Thus, the true role of ITCH in GC is still controversial and further studies will be necessary to identify the downstream substrates and mechanisms.
WWP1
WW domain-containing E3 ubiquitin protein ligase 1 (WWP1, also known as AIP5 or TIUL1) is related to NEDD4 family members, contains four WW domains, and is primarily involved in inflammation, neurological disorders, cardiovascular diseases, and malignancies (76) (Figure 1). Elevated expression of WWP1 has been detected in human GC tissues and cells, and has been shown to be associated with poor clinicopathological characteristics and worse survival (26). Besides, downregulation of WWP1 leads to compromised tumor proliferation, as well as enhanced G0/G1-phase arrest and apoptosis by governing the PTEN/AKT signaling pathway (26). Its similar oncogenic activity has also been confirmed in subsequent studies, evidenced by the fact that WWP1 is a direct target of miR-584-5p, miR-129-5p, and miR-129-3p, and these miRNAs inhibit GC tumor growth by targeting WWP1 (27,28). All of these studies indicate that WWP1 is an important tumor promoter and promising target in GC.
WWP2
WWP2 (also known as AIP2) functions as an E3 to regulate a myriad of cellular activities (77), which is interestingly emerging to have a role in carcinogenesis. At present, only one study has provided insight into the role of WWP2 in GC. WWP2 was found to be upregulated in GC tissues compared with paired adjacent non-tumor tissues (29). In patients with GC, the upregulated expression of WWP2 contributed to an unfavorable prognosis (29). In addition, depletion of WWP2 increases PTEN protein levels and decreases AKT phosphorylation level, thereby inhibiting the proliferation of GC cells both in vitro and in vivo (29). However, whether WWP2 regulates PTEN/PI3K/AKT pathway through its E3 ligase activity remains elusive.
SMURF1
Like other NEDD4 family members, SMAD-specific E3 ubiquitin protein ligase 1 (SMURF1) interacts with many potential targets and plays a role in different cellular functions including bone homeostasis, embryogenesis, autophagy, and carcinogenesis (78). Deregulated expression of SMURF1 has been reported in several human cancers, and its increased expression is frequently associated with disease progression and prognosis (79). Interestingly, SMURF1 appears to have a dual role in carcinogenesis, the majority of the studies assign SMURF1 a role of oncogenic factor. In GC, highly expressed SMURF1 has been observed in tumor tissues and cell lines, which was associated to malignant phenotypes and decreased survival (30). The pro-tumor property of SMURF1 was attributed to suppress the expression of DAB2IP and subsequently enhances activation of the PI3K/AKT pathway (30). Besides, SMURF1 has been reported to be a target of miR-424, thereby regulating cisplatin-resistant advanced GC (31). Similarly, Jiang et al. have unveiled that miR-1254 could target the 3′-UTR of SMURF1 and inhibit its protein expression to inactivate the PI3K/AKT pathway, thus repressing the proliferation, migration, invasion, and EMT of GC cells (32). Together, the above results point to SMURF1 as an activator of the PI3K/AKT pathway and suggest that it functions as a tumor promoter in GC. Interestingly, SMURF1 mediated pluripotency degradation of MEKK2 was attenuated by the interaction of Kir2.1 with STK38, which resulted in activation of MEK1/2–ERK1/2 signaling also stimulating GC cell invasion and metastasis (33).
Other HECT E3 ligases
HUWE1
The HECT, UBA, and WWE domain-containing E3 ubiquitin protein ligase 1 (HUWE1, also known as Mule, HectH9, ARF-BP1, HSPC272, Ib772, URE-B1, E3Histone, and LASU1) is a large and evolutionarily conserved E3 belonging to the other HECT subfamily (64) (Figure 1). In most cases, HUWE1 could regulate many biological processes, such as DNA damage repair, transcription, cell proliferation, differentiation, autophagy, and apoptosis (80). Available evidence shows that HUWE1 is interestingly emerging to have a role in tumorigenesis, and in GC as well. Very little was known about how HUWE1 regulates GC, until recently, when He et al. reported the role and mechanistic studies of HUWE1 in GC. The results showed that GC patients expressed high levels of HUWE1 and low protein levels of TGFBR2 (34). In addition, overexpression of HUWE1 promoted the proliferative activity, migratory, and invasive potential of GC cells (34). Mechanistically, HUWE1 can degrade the tumor suppressor TGFBR2 through ubiquitination, leading to the malignant progression of GC (34). The extent of HUWE1 contribution to gastric malignancies is still far from being understood.
HACE1
In addition to HECT domain, the E3 ligase HACE1 harbors six ankyrin repeats that function to mediate protein-protein interactions (Figure 1). HACE1 has attracted much attention in recent years because of its involvement in the development of human malignancies, in which it acts as a tumor suppressor (81). Chen et al. found that HACE1 was significantly reduced in GC tissues and cell lines, and it was closely correlated with tumor pathological differentiation (35). Accordingly, lower HACE1 expression is associated with better overall survival rate in TNM stages I-IIIa GC patients (35). In addition, overexpression of HACE1 suppressed the ability of proliferation and migration of GC cells, and enhanced cell apoptosis (35). Mechanistically, HACE1 can downregulate the expression of β-catenin, which in turn inhibits the activity of the Wnt signal to impede the malignant progression of GC (35). Furthermore, the authors demonstrated HACE1 executes its regulation of the Wnt/β-catenin signaling through its E3 ligase activity (35). However, the specific substrates in the Wnt/β-catenin signaling of HACE1 in GC are still largely unknown. Besides, HACE1 has been reported to cause ubiquitination of cyclin C in the non-proteolytic K11 linkage form, which led to the cisplatin-induced nuclear–mitochondrial translocation of cyclin C and finally promoted apoptosis of GC cells (36). The investigation of Sakata M et al. showed that hypermethylation of the HACE1 gene is associated with reduced expression of HACE1 (37), which points out that demethylation of HACE1 may be a new strategy for the treatment of GC.
UBR5
The other HECT E3 ligase UBR5 (also known as EDD1, EDD, DD5, HYD, or KIAA0896), consists of a ubiquitin-binding UBA domain, a zinc finger-like UBR domain involved in N-rule substrate recognition, two nuclear localization sequences, a PABC/MLLE domain for protein–protein interaction, and a C-terminal HECT (82) (Figure 1). UBR5 has been related to the regulation of DNA damage response, translation, metabolism, transcription, and apoptosis (83). Recent evidence has unveiled a critical role of UBR5 (38)in the progression of multiple cancer types, including GC. UBR5 was mutated in 27.8% of GC, and GC patients with high UBR5 expression had a poor prognosis (39,40). Moreover, Knockdown of UBR5 in GC cells was shown to suppress cell growth, migration and invasion, which is mainly due to the inhibition of protein stability of tumor suppressor GKN1 through ubiquitination (84).
UBE3C
UBE3C, also known as RAUL, contains an N-terminal IQ motif and a C-terminal HECT domain (Figure 1), and is able to assemble K48 and less preponderant K29 and K11 linkages (85). A study identified the N-terminal region just preceding the HECT domain is crucial for the stabilization and enzymatic activity of UBE3C (86). Accumulating evidence indicated that UBE3C is implicated in several tumors, including glioma, melanoma, renal cell carcinoma, hepatocellular carcinoma, breast cancer, lung cancer, and gastric cancer (41,42,87-91). And the role of UBE3C in gastric cancer has only recently begun to be explored. Zhao et al. discovered that LINC00355, as a tumor promoter, promotes the malignant progression of GC by enhancing the association of E3 ubiquitin ligases UBE3C and RAD18 with P53, facilitating its ubiquitination and degradation (43). Furthermore, another study identified that the protein and mRNA levels of UBE3C in GC were both heightened and inversely correlated with patient outcomes. UBE3C could interact directly with and downregulate AXIN1, thus activating β-catenin signaling and promoting the development and progression of GC (42).
HECTD3
Homologous to the E6-associated protein carboxyl terminus domain containing 3 (HECTD3) is an under-investigated other HECT E3 ligase and characterized by an N-terminal DOC domain (Figure 1). Recently, Zhang et al. discovered the abnormal activation of HECTD3 in GC cells, which was correlated with poor outcomes of patients (92). Also, HECTD3 could facilitate the malignant proliferation and tumorigenesis of GC cells, and inhibit the cell apoptosis, suggesting that it serves as a tumor promoter in GC. Mechanistically, HECTD can stabilize expression of c-MYC by interacting with c-MYC and mediating K29 linked polyubiquitination of c-MYC (92).
Conclusion and perspective
The data reviewed here illustrate genetic alteration, abnormal expression, or dysfunction of HECT E3 ligases that may contribute to GC occurrence and progression (Table 1; Figure 2). However, several HECT E3 ligases have been reported to play a dual role in GC (Table 1). The concrete mechanisms have not been fully explored. Currently, E3 ubiquitin ligases become important therapeutic targets for cancer treatment (10,93). Many inhibitors of the RING family E3 ligases have been developed for cancer therapy, while only limited efforts have been made regarding the HECT E3 ligases. With the better understanding of the mechanism underlying regulation of the HECT E3 ubiquitin ligases, and advances in research technology, such as high-throughput screenings and PROTAC (proteolysis targeting chimera) technology, we will be able to develop more drugs that target the HECT E3 ligases for GC therapy.
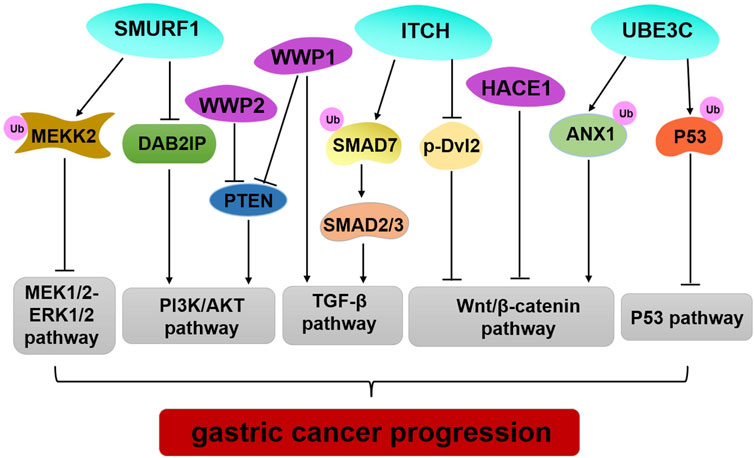
FIGURE 2. Regulation of important signaling pathways by HECT E3 ligases in GC. SMURF1 mediated pluripotency degradation of MEKK2, which resulted in inactivation of MEK1/2–ERK1/2 signaling. SMURF1, WWP1, and WWP2 have similar functions by regulating PIAK/AKT signaling pathway. Limiting ITCH activity causes accumulation of SMAD7 and blocks TGF- β1 signal transduction to inhibit GC progression. ITCH also exerts tumor-suppressive functions by down-regulating the protein level of β-catenin and p-Dvl2. HACE1 executes its regulation of the Wnt/β-catenin signaling pathway through its E3 ligase activity. UBE3C promotes ubiquitination and degradation ANX1 to activate the WNT/β-catenin signaling in GC development. UBE3C directly binds to p53, and it mediates p53 degradation to inhibit the p53 pathway in GC.
Author contributions
AS, WY, and QL wrote this manuscript. XT and YC prepared the figures and tables. All authors contributed to the article and approved the final version of the manuscript.
Funding
This work was supported by the National Natural Science Foundation of China (Nos. 82002581, 81871888, and 82172942), China Postdoctoral Science Foundation (No. 2020M671375), Jiangsu Province Postdoctoral Research Funding Scheme (No. 2020Z261).
Conflict of interest
The authors declare that the research was conducted in the absence of any commercial or financial relationships that could be construed as a potential conflict of interest.
References
1. Hershko, A. Ubiquitin: Roles in protein modification and breakdown. Cell (1983) 34:11–2. doi:10.1016/0092-8674(83)90131-9
2. Pickart, CM. Mechanisms underlying ubiquitination. Annu Rev Biochem (2001) 70:503–33. doi:10.1146/annurev.biochem.70.1.503
3. Rape, M. Ubiquitylation at the crossroads of development and disease. Nat Rev Mol Cel Biol (2018) 19:59–70. doi:10.1038/nrm.2017.83
4. Kwon, YT, and Ciechanover, A. The ubiquitin code in the ubiquitin-proteasome system and autophagy. Trends Biochemical Sciences (2017) 42:873–86. doi:10.1016/j.tibs.2017.09.002
5. Ciechanover, A. The unravelling of the ubiquitin system. Nat Rev Mol Cel Biol (2015) 16:322–4. doi:10.1038/nrm3982
6. Schulman, BA, and Harper, JW. Ubiquitin-like protein activation by E1 enzymes: The apex for downstream signalling pathways. Nat Rev Mol Cel Biol (2009) 10:319–31. doi:10.1038/nrm2673
7. Ye, Y, and Rape, M. Building ubiquitin chains: E2 enzymes at work. Nat Rev Mol Cel Biol (2009) 10:755–64. doi:10.1038/nrm2780
8. Yang, Q, Zhao, J, Chen, D, and Wang, Y. E3 ubiquitin ligases: Styles, structures and functions. Mol Biomed (2021) 2:23. doi:10.1186/s43556-021-00043-2
9. Buetow, L, and Huang, DT. Structural insights into the catalysis and regulation of E3 ubiquitin ligases. Nat Rev Mol Cel Biol (2016) 17:626–42. doi:10.1038/nrm.2016.91
10. Deng, L, Meng, T, Chen, L, Wei, W, and Wang, P. The role of ubiquitination in tumorigenesis and targeted drug discovery. Signal Transduction Targeted Therapy (2020) 5:11. doi:10.1038/s41392-020-0107-0
11. Senft, D, Qi, J, and Ronai, ZA. Ubiquitin ligases in oncogenic transformation and cancer therapy. Nat Rev Cancer (2018) 18:69–88. doi:10.1038/nrc.2017.105
12. Sluimer, J, and Distel, B. Regulating the human HECT E3 ligases. Cell Mol Life Sci : CMLS (2018) 75:3121–41. doi:10.1007/s00018-018-2848-2
13. Sung, H, Ferlay, J, Siegel, RL, Laversanne, M, Soerjomataram, I, Jemal, A, et al. Global cancer statistics 2020: GLOBOCAN estimates of incidence and mortality worldwide for 36 cancers in 185 countries. CA: a Cancer J clinicians (2021) 71:209–49. doi:10.3322/caac.21660
14. Arnold, M, Abnet, CC, Neale, RE, Vignat, J, Giovannucci, EL, McGlynn, KA, et al. Global burden of 5 major types of gastrointestinal cancer. Gastroenterology (2020) 159:335–49. doi:10.1053/j.gastro.2020.02.068
15. Shi, J, Qu, YP, and Hou, P. Pathogenetic mechanisms in gastric cancer. World J Gastroenterol (2014) 20:13804–19. doi:10.3748/wjg.v20.i38.13804
16. Shimizu, D, Kanda, M, and Kodera, Y. Review of recent molecular landscape knowledge of gastric cancer. Histology and histopathology (2018) 33:11–26. doi:10.14670/hh-11-898
17. Kim, SS, Yoo, NJ, Jeong, EG, Kim, MS, and Lee, SH. Expression of NEDD4-1, a PTEN regulator, in gastric and colorectal carcinomas. APMIS : Acta pathologica, microbiologica, immunologica Scand (2008) 116:779–84. doi:10.1111/j.1600-0463.2008.00999.x
18. Wang, YY, Ye, ZY, Zhao, ZS, Tao, HQ, and Li, SG. Systems biology approach to identification of biomarkers for metastatic progression in gastric cancer. J Cancer Res Clin Oncol (2010) 136:135–41. doi:10.1007/s00432-009-0644-y
19. Sun, A, Yu, G, Dou, X, Yan, X, Yang, W, and Lin, Q. Nedd4-1 is an exceptional prognostic biomarker for gastric cardia adenocarcinoma and functionally associated with metastasis. Mol Cancer (2014) 13:248. doi:10.1186/1476-4598-13-248
20. Yang, Z, Yuan, XG, Chen, J, and Lu, NH. Is NEDD4-1 a negative regulator of phosphatase and tensin homolog in gastric carcinogenesis? World J Gastroenterol (2012) 18:6345–8. doi:10.3748/wjg.v18.i43.6345
21. Gao, C, Pang, L, Ren, C, and Ma, T. Decreased expression of Nedd4L correlates with poor prognosis in gastric cancer patient. Med Oncol (Northwood, Lond England) (2012) 29:1733–8. doi:10.1007/s12032-011-0061-3
22. Jiang, X, Zhang, S, Yin, Z, Sheng, Y, Yan, Q, Sun, R, et al. The correlation between NEDD4L and HIF-1α levels as a gastric cancer prognostic marker. Int J Med Sci (2019) 16:1517–24. doi:10.7150/ijms.34646
23. Gen, Y, Yasui, K, Kitaichi, T, Iwai, N, Terasaki, K, Dohi, O, et al. ASPP2 suppresses invasion and TGF-β1-induced epithelial-mesenchymal transition by inhibiting Smad7 degradation mediated by E3 ubiquitin ligase ITCH in gastric cancer. Cancer Lett (2017) 398:52–61. doi:10.1016/j.canlet.2017.04.002
24. Peng, Y, and Wang, HH. Cir-ITCH inhibits gastric cancer migration, invasion and proliferation by regulating the Wnt/β-catenin pathway. Scientific Rep (2020) 10:17443. doi:10.1038/s41598-020-74452-8
25. Ghasemi, S, Emadi-Baygi, M, and Nikpour, P. Down-regulation of circular RNA ITCH and circHIPK3 in gastric cancer tissues. Turkish J Med Sci (2019) 49:687–95. doi:10.3906/sag-1806-50
26. Zhang, L, Wu, Z, Ma, Z, Liu, H, Wu, Y, and Zhang, Q. WWP1 as a potential tumor oncogene regulates PTEN-Akt signaling pathway in human gastric carcinoma. Tumour Biol : J Int Soc Oncodevelopmental Biol Med (2015) 36:787–98. doi:10.1007/s13277-014-2696-0
27. Li, Q, Li, Z, Wei, S, Wang, W, Chen, Z, Zhang, L, et al. Overexpression of miR-584-5p inhibits proliferation and induces apoptosis by targeting WW domain-containing E3 ubiquitin protein ligase 1 in gastric cancer. J Exp Clin Cancer Res : CR (2017) 36:59. doi:10.1186/s13046-017-0532-2
28. Ma, L, Chen, X, Li, C, Cheng, R, Gao, Z, Meng, X, et al. miR-129-5p and -3p co-target WWP1 to suppress gastric cancer proliferation and migration. J Cell Biochem (2018) 120:7527–38. doi:10.1002/jcb.28027
29. Wang, K, Liu, J, Zhao, X, Li, H, Luo, G, Yu, Y, et al. WWP2 regulates proliferation of gastric cancer cells in a PTEN-dependent manner. Biochem biophysical Res Commun (2020) 521:652–9. doi:10.1016/j.bbrc.2019.10.179
30. Tao, Y, Sun, C, Zhang, T, and Song, Y. SMURF1 promotes the proliferation, migration and invasion of gastric cancer cells. Oncol Rep (2017) 38:1806–14. doi:10.3892/or.2017.5825
31. Lu, L, Wu, M, Lu, Y, Zhao, Z, Liu, T, Fu, W, et al. MicroRNA-424 regulates cisplatin resistance of gastric cancer by targeting SMURF1 based on GEO database and primary validation in human gastric cancer tissues. OncoTargets Ther (2019) 12:7623–36. doi:10.2147/ott.S208275
32. Jiang, M, Shi, L, Yang, C, Ge, Y, Lin, L, Fan, H, et al. miR-1254 inhibits cell proliferation, migration, and invasion by down-regulating Smurf1 in gastric cancer. Cel Death Dis (2019) 10:32. doi:10.1038/s41419-018-1262-x
33. Ji, CD, Wang, YX, Xiang, DF, Liu, Q, Zhou, ZH, Qian, F, et al. Kir2.1 interaction with Stk38 promotes invasion and metastasis of human gastric cancer by enhancing MEKK2-MEK1/2-ERK1/2 signaling. Cancer Res (2018) 78:3041–53. doi:10.1158/0008-5472.Can-17-3776
34. He, Y, Zhou, J, and Wan, Q. The E3 ligase HUWE1 mediates TGFBR2 ubiquitination and promotes gastric cancer cell proliferation, migration, and invasion. Invest New Drugs (2021) 39:713–23. doi:10.1007/s10637-020-01041-x
35. Chen, YL, Li, DP, Jiang, HY, Yang, Y, Xu, LL, Zhang, SC, et al. Overexpression of HACE1 in gastric cancer inhibits tumor aggressiveness by impeding cell proliferation and migration. Cancer Med (2018) 7:2472–84. doi:10.1002/cam4.1496
36. Jiang, HY, Chen, YL, Xu, XX, Li, CY, Chen, Y, Li, DP, et al. Ubiquitylation of cyclin C by HACE1 regulates cisplatin-associated sensitivity in gastric cancer. Clin translational Med (2022) 12:e770. doi:10.1002/ctm2.770
37. Sakata, M, Kitamura, YH, Sakuraba, K, Goto, T, Mizukami, H, Saito, M, et al. Methylation of HACE1 in gastric carcinoma. Anticancer Res (2009) 29:2231–3.
38. Adhikary, S, Marinoni, F, Hock, A, Hulleman, E, Popov, N, Beier, R, et al. The ubiquitin ligase HectH9 regulates transcriptional activation by Myc and is essential for tumor cell proliferation. Cell (2005) 123:409–21. doi:10.1016/j.cell.2005.08.016
39. Kim, MS, Oh, JE, Eom, HS, Yoo, NJ, and Lee, SH. Mutational analysis of UBR5 gene encoding an E3 ubiquitin ligase in common human cancers. Pathology (2010) 42:93–4. doi:10.3109/00313020903434322
40. Ding, F, Zhu, X, Song, X, Yuan, P, Ren, L, Chai, C, et al. UBR5 oncogene as an indicator of poor prognosis in gastric cancer. Exp Ther Med (2020) 20:7. doi:10.3892/etm.2020.9135
41. Gu, J, Mao, W, Ren, W, Xu, F, Zhu, Q, Lu, C, et al. Ubiquitin-protein ligase E3C maintains non-small-cell lung cancer stemness by targeting AHNAK-p53 complex. Cancer Lett (2019) 443:125–34. doi:10.1016/j.canlet.2018.11.029
42. Zhang, Y, Xu, J, Fu, H, Wei, Z, Yang, D, and Yan, R. UBE3C promotes proliferation and inhibits apoptosis by activating the β-catenin signaling via degradation of AXIN1 in gastric cancer. Carcinogenesis (2021) 42:285–93. doi:10.1093/carcin/bgaa098
43. Zhao, W, Jin, Y, Wu, P, Yang, J, Chen, Y, Yang, Q, et al. LINC00355 induces gastric cancer proliferation and invasion through promoting ubiquitination of P53. Cel Death Discov (2020) 6:99. doi:10.1038/s41420-020-00332-9
44. Rotin, D, and Kumar, S. Physiological functions of the HECT family of ubiquitin ligases. Nat Rev Mol Cel Biol (2009) 10:398–409. doi:10.1038/nrm2690
45. Berndsen, CE, and Wolberger, C. New insights into ubiquitin E3 ligase mechanism. Nat Struct Mol Biol (2014) 21:301–7. doi:10.1038/nsmb.2780
46. Scheffner, M, and Kumar, S. Mammalian HECT ubiquitin-protein ligases: Biological and pathophysiological aspects. Biochim Biophys Acta (2014) 1843:61–74. doi:10.1016/j.bbamcr.2013.03.024
47. Deshaies, RJ, and Joazeiro, CA. RING domain E3 ubiquitin ligases. Annu Rev Biochem (2009) 78:399–434. doi:10.1146/annurev.biochem.78.101807.093809
48. Metzger, MB, Pruneda, JN, Klevit, RE, and Weissman, AM. RING-Type E3 ligases: Master manipulators of E2 ubiquitin-conjugating enzymes and ubiquitination. Biochim Biophys Acta (2014) 1843:47–60. doi:10.1016/j.bbamcr.2013.05.026
49. Spratt, DE, Walden, H, and Shaw, GS. RBR E3 ubiquitin ligases: New structures, new insights, new questions. Biochem J (2014) 458:421–37. doi:10.1042/bj20140006
50. Walden, H, and Rittinger, K. RBR ligase-mediated ubiquitin transfer: A tale with many twists and turns. Nat Struct Mol Biol (2018) 25:440–5. doi:10.1038/s41594-018-0063-3
51. Zheng, N, and Shabek, N. Ubiquitin ligases: Structure, function, and regulation. Annu Rev Biochem (2017) 86:129–57. doi:10.1146/annurev-biochem-060815-014922
52. Wang, Y, Argiles-Castillo, D, Kane, EI, Zhou, A, and Spratt, DE. HECT E3 ubiquitin ligases - emerging insights into their biological roles and disease relevance. J Cel Sci (2020) 133:jcs228072. doi:10.1242/jcs.228072
53. Zou, X, Levy-Cohen, G, and Blank, M. Molecular functions of NEDD4 E3 ubiquitin ligases in cancer. Biochim Biophys Acta (2015) 1856:91–106. doi:10.1016/j.bbcan.2015.06.005
54. Plant, PJ, Yeger, H, Staub, O, Howard, P, and Rotin, D. The C2 domain of the ubiquitin protein ligase Nedd4 mediates Ca2+-dependent plasma membrane localization. J Biol Chem (1997) 272:32329–36. doi:10.1074/jbc.272.51.32329
55. Yamaguchi, K, Ohara, O, Ando, A, and Nagase, T. Smurf1 directly targets hPEM-2, a GEF for Cdc42, via a novel combination of protein interaction modules in the ubiquitin-proteasome pathway. Biol Chem (2008) 389:405–13. doi:10.1515/bc.2008.036
56. Tian, M, Bai, C, Lin, Q, Lin, H, Liu, M, Ding, F, et al. Binding of RhoA by the C2 domain of E3 ligase Smurf1 is essential for Smurf1-regulated RhoA ubiquitination and cell protrusive activity. FEBS Lett (2011) 585:2199–204. doi:10.1016/j.febslet.2011.06.016
57. Wiesner, S, Ogunjimi, AA, Wang, HR, Rotin, D, Sicheri, F, Wrana, JL, et al. Autoinhibition of the HECT-type ubiquitin ligase Smurf2 through its C2 domain. Cell (2007) 130:651–62. doi:10.1016/j.cell.2007.06.050
58. Dunn, R, Klos, DA, Adler, AS, and Hicke, L. The C2 domain of the Rsp5 ubiquitin ligase binds membrane phosphoinositides and directs ubiquitination of endosomal cargo. J Cel Biol (2004) 165:135–44. doi:10.1083/jcb.200309026
59. Dodson, EJ, Fishbain-Yoskovitz, V, Rotem-Bamberger, S, and Schueler-Furman, O. Versatile communication strategies among tandem WW domain repeats. Exp Biol Med (Maywood, N.J.) (2015) 240:351–60. doi:10.1177/1535370214566558
60. Bork, P, and Sudol, M. The WW domain: A signalling site in dystrophin? Trends Biochemical Sciences (1994) 19:531–3. doi:10.1016/0968-0004(94)90053-1
61. Staub, O, Dho, S, Henry, P, Correa, J, Ishikawa, T, McGlade, J, et al. WW domains of Nedd4 bind to the proline-rich PY motifs in the epithelial Na+ channel deleted in Liddle's syndrome. EMBO J (1996) 15:2371–80. doi:10.1002/j.1460-2075.1996.tb00593.x
62. Nemergut, ME, Mizzen, CA, Stukenberg, T, Allis, CD, and Macara, IG. Chromatin docking and exchange activity enhancement of RCC1 by histones H2A and H2B. Science (New York, N.Y.) (2001) 292:1540–3. doi:10.1126/science.292.5521.1540
63. Zhang, C, and Clarke, PR. Chromatin-independent nuclear envelope assembly induced by Ran GTPase in Xenopus egg extracts. Science (New York, N.Y.) (2000) 288:1429–32. doi:10.1126/science.288.5470.1429
64. Singh, S, Ng, J, and Sivaraman, J. Exploring the "Other" subfamily of HECT E3-ligases for therapeutic intervention. Pharmacol Ther (2021) 224:107809. doi:10.1016/j.pharmthera.2021.107809
65. Grau-Bové, X, Sebé-Pedrós, A, and Ruiz-Trillo, I. A genomic survey of HECT ubiquitin ligases in eukaryotes reveals independent expansions of the HECT system in several lineages. Genome Biol Evol (2013) 5:833–47. doi:10.1093/gbe/evt052
66. Marín, I. Animal HECT ubiquitin ligases: Evolution and functional implications. BMC Evol Biol (2010) 10:56. doi:10.1186/1471-2148-10-56
67. Wang, ZW, Hu, X, Ye, M, Lin, M, Chu, M, and Shen, X. NEDD4 E3 ligase: Functions and mechanism in human cancer. Semin Cancer Biol (2020) 67:92–101. doi:10.1016/j.semcancer.2020.03.006
68. Yang, B, and Kumar, S. Nedd4 and nedd4-2: Closely related ubiquitin-protein ligases with distinct physiological functions. Cel Death Differ (2010) 17:68–77. doi:10.1038/cdd.2009.84
69. Gao, S, Alarcón, C, Sapkota, G, Rahman, S, Chen, PY, Goerner, N, et al. Ubiquitin ligase Nedd4L targets activated Smad2/3 to limit TGF-beta signaling. Mol Cel (2009) 36:457–68. doi:10.1016/j.molcel.2009.09.043
70. Wang, Z, Dang, T, Liu, T, Chen, S, Li, L, Huang, S, et al. NEDD4L protein catalyzes ubiquitination of PIK3CA protein and regulates PI3K-AKT signaling. J Biol Chem (2016) 291:17467–77. doi:10.1074/jbc.M116.726083
71. Ding, Y, Zhang, Y, Xu, C, Tao, QH, and Chen, YG. HECT domain-containing E3 ubiquitin ligase NEDD4L negatively regulates Wnt signaling by targeting dishevelled for proteasomal degradation. J Biol Chem (2013) 288:8289–98. doi:10.1074/jbc.M112.433185
72. Chan, W, Tian, R, Lee, YF, Sit, ST, Lim, L, and Manser, E. Down-regulation of active ACK1 is mediated by association with the E3 ubiquitin ligase Nedd4-2. J Biol Chem (2009) 284:8185–94. doi:10.1074/jbc.M806877200
73. Melino, G, Gallagher, E, Aqeilan, RI, Knight, R, Peschiaroli, A, Rossi, M, et al. Itch: A HECT-type E3 ligase regulating immunity, skin and cancer. Cel Death Differ (2008) 15:1103–12. doi:10.1038/cdd.2008.60
74. Lallemand, F, Seo, SR, Ferrand, N, Pessah, M, L'Hoste, S, Rawadi, G, et al. AIP4 restricts transforming growth factor-beta signaling through a ubiquitination-independent mechanism. J Biol Chem (2005) 280:27645–53. doi:10.1074/jbc.M500188200
75. Park, SH, Jung, EH, Kim, GY, Kim, BC, Lim, JH, and Woo, CH. Itch E3 ubiquitin ligase positively regulates TGF-β signaling to EMT via Smad7 ubiquitination. Mol Cell (2015) 38:20–5. doi:10.14348/molcells.2015.2120
76. Zhi, X, and Chen, C. WWP1: A versatile ubiquitin E3 ligase in signaling and diseases. Cell Mol Life Sci : CMLS (2012) 69:1425–34. doi:10.1007/s00018-011-0871-7
77. Chen, W, Jiang, X, and Luo, Z. WWP2: A multifunctional ubiquitin ligase gene. Pathol Oncol Res : POR (2014) 20:799–803. doi:10.1007/s12253-014-9838-y
78. Cao, Y, and Zhang, L. A Smurf1 tale: Function and regulation of an ubiquitin ligase in multiple cellular networks. Cell Mol Life Sci : CMLS (2013) 70:2305–17. doi:10.1007/s00018-012-1170-7
79. Fu, L, Cui, CP, Zhang, X, and Zhang, L. The functions and regulation of Smurfs in cancers. Semin Cancer Biol (2020) 67:102–16. doi:10.1016/j.semcancer.2019.12.023
80. Qi, L, Xu, X, and Qi, X. The giant E3 ligase HUWE1 is linked to tumorigenesis, spermatogenesis, intellectual disability, and inflammatory diseases. Front Cell Infect Microbiol (2022) 12:905906. doi:10.3389/fcimb.2022.905906
81. Zhang, L, Anglesio, MS, O'Sullivan, M, Zhang, F, Yang, G, Sarao, R, et al. The E3 ligase HACE1 is a critical chromosome 6q21 tumor suppressor involved in multiple cancers. Nat Med (2007) 13:1060–9. doi:10.1038/nm1621
82. Matta-Camacho, E, Kozlov, G, Menade, M, and Gehring, K. Structure of the HECT C-lobe of the UBR5 E3 ubiquitin ligase. Acta Crystallographica Section F, Struct Biol crystallization Commun (2012) 68:1158–63. doi:10.1107/s1744309112036937
83. Shearer, RF, Iconomou, M, Watts, CK, and Saunders, DN. Functional roles of the E3 ubiquitin ligase UBR5 in cancer. Mol Cancer Res : MCR (2015) 13:1523–32. doi:10.1158/1541-7786.Mcr-15-0383
84. Yang, M, Jiang, N, Cao, QW, Ma, MQ, and Sun, Q. The E3 ligase UBR5 regulates gastric cancer cell growth by destabilizing the tumor suppressor GKN1. Biochem biophysical Res Commun (2016) 478:1624–9. doi:10.1016/j.bbrc.2016.08.170
85. Michel, MA, Elliott, PR, Swatek, KN, Simicek, M, Pruneda, JN, Wagstaff, JL, et al. Assembly and specific recognition of k29-and k33-linked polyubiquitin. Mol Cel (2015) 58:95–109. doi:10.1016/j.molcel.2015.01.042
86. Singh, S, and Sivaraman, J. Crystal structure of HECT domain of UBE3C E3 ligase and its ubiquitination activity. Biochem J (2020) 477:905–23. doi:10.1042/bcj20200027
87. Pan, SJ, Zhan, SK, Ji, WZ, Pan, YX, Liu, W, Li, DY, et al. Ubiquitin-protein ligase E3C promotes glioma progression by mediating the ubiquitination and degrading of Annexin A7. Scientific Rep (2015) 5:11066. doi:10.1038/srep11066
88. Tang, L, Yi, XM, Chen, J, Chen, FJ, Lou, W, Gao, YL, et al. Ubiquitin ligase UBE3C promotes melanoma progression by increasing epithelial-mesenchymal transition in melanoma cells. Oncotarget (2016) 7:15738–46. doi:10.18632/oncotarget.7393
89. Wen, JL, Wen, XF, Li, RB, Jin, YC, Wang, XL, Zhou, L, et al. UBE3C promotes growth and metastasis of renal cell carcinoma via activating Wnt/β-catenin pathway. PloS one (2015) 10:e0115622. doi:10.1371/journal.pone.0115622
90. Jiang, JH, Liu, YF, Ke, AW, Gu, FM, Yu, Y, Dai, Z, et al. Clinical significance of the ubiquitin ligase UBE3C in hepatocellular carcinoma revealed by exome sequencing. Hepatol (Baltimore, Md (2014) 59:2216–27. doi:10.1002/hep.27012
91. Xiong, J, Wei, B, Ye, Q, and Liu, W. MiR-30a-5p/UBE3C axis regulates breast cancer cell proliferation and migration. Biochem biophysical Res Commun (2019) 516:1013–8. doi:10.1016/j.bbrc.2016.03.069
92. Zhang, G, Zhu, Q, Yan, X, Ci, M, Zhao, E, Hou, J, et al. HECTD3 promotes gastric cancer progression by mediating the polyubiquitination of c-MYC. Cel Death Discov (2022) 8:185. doi:10.1038/s41420-022-01001-9
Keywords: gastric cancer, ubiquitination, tumor suppressor, HECT E3 ubiquitin ligases, oncoprotein
Citation: Sun A, Tian X, Chen Y, Yang W and Lin Q (2023) Emerging roles of the HECT E3 ubiquitin ligases in gastric cancer. Pathol. Oncol. Res. 29:1610931. doi: 10.3389/pore.2023.1610931
Received: 07 November 2022; Accepted: 16 January 2023;
Published: 07 February 2023.
Edited by:
Anna Sebestyén, Semmelweis University, HungaryCopyright © 2023 Sun, Tian, Chen, Yang and Lin. This is an open-access article distributed under the terms of the Creative Commons Attribution License (CC BY). The use, distribution or reproduction in other forums is permitted, provided the original author(s) and the copyright owner(s) are credited and that the original publication in this journal is cited, in accordance with accepted academic practice. No use, distribution or reproduction is permitted which does not comply with these terms.
*Correspondence: Aiqin Sun, YWlxaW5zdW5AdWpzLmVkdS5jbg==; Qiong Lin, cWxpbkB1anMuZWR1LmNu