- 1Department of Surgical and Molecular Pathology, National Tumour Biology Laboratory, National Institute of Oncology, Budapest, Hungary
- 2National Koranyi Institute of Pulmonology, Budapest, Hungary
- 3Faculty of Medicine, University of Pécs, Pécs, Hungary
- 4Department of Oncology Faculty of Medicine, Semmelweis University, Budapest, Hungary
EGFR mutation in non-small cell lung cancer (NSCLC) offers a potential therapeutic target for tyrosine kinase inhibitor (TKI) therapy. The majority of these cases, however eventually develop therapy resistance, mainly by acquiring EGFR T790M mutation. Recently, third-generation TKIs have been introduced to overcome T790M mutation-related resistance. Cell free circulating tumor DNA (liquid biopsy) has emerged as a valuable alternative method for T790M mutation detection during patient follow up, when a tissue biopsy cannot be obtained for analysis. In this study, we summarized our experience with Super-ARMS EGFR Mutation Detection Kit (AmoyDx) on 401 samples of 242 NSCLC patients in a 3-year period in Hungary, comprising 364 plasma and 37 non-plasma samples. We also compared the performance of two commercially available detection kits, the cobas EGFR Mutation test v2 (Roche) and the Super-ARMS EGFR Mutation Detection Kit (AmoyDx). The same activating EGFR mutation was detected with the AmoyDx kit as in the primary tumor in 45.6% of the samples. T790M mutation was identified in 48.1% of the samples containing activating EGFR mutation. The detection rate of T790M mutation was not dependent on the DNA concentration of the plasma sample and there was no considerable improvement in mutation detection rate after a second, subsequent plasma sample. The concordance of EGFR activating mutation detection was 89% between the two methods, while this was 93% for T790M mutation detection. The AmoyDx kit, however showed an overall higher detection rate of T790M mutation compared to the cobas kit (p = 0.014). T790M mutation was detected at 29.8% of the patients if only plasma samples were available for analysis, while the detection rate was 70.2% in non-plasma samples. If the activating EGFR was detected in the plasma samples, the detection rate of T790M mutation was 42.4%. Although non-plasma samples provided a superior T790M mutation detection rate, we found that liquid biopsy can offer a valuable tool for T790M mutation detection, when a tissue biopsy is not available. Alternatively, a liquid biopsy can be used as a screening test, when re-biopsy should be considered in case of wild-type results.
Introduction
Lung cancer is one of the most common malignant tumors with high mortality rate. Hungary was reported to have one of the highest incidence and mortality rates of lung cancer worldwide (1). The majority of the patients are smokers. In European studies, mutation in the EGFR gene exon 18, 19, 20 or 21 can be detected in 12%–17% of non-small cell lung cancers (NSCLC). These patients are predominantly non-smokers with a diagnosis of adenocarcinoma (2). EGFR tyrosine kinase inhibitor (TKI) therapy proved to be highly effective in these cases. The most common activating mutations are the point mutation L858R and a subset of in-frame deletions involving exon 19 (3). While the first-generation TKIs, such as gefitinib and erlotinib reversibly bind to EGFR, the binding and inhibition are irreversible at second-generation inhibitors, such as afatinib and dacomitinib. Despite this difference in their tyrosine-kinase binding ability, there is a similar pattern of acquired resistance, which usually occurs after 9–14 months (4). The resistance mechanism mainly involves gatekeeper EGFR mutations, activation of alternative signaling pathways through MET and ERBB2 amplifications or activation of downstream MAPK or PI3K pathways (5). Among secondary gatekeeper EGFR mutations, T790M substitution mutation in exon 20 has emerged as the most common mechanism of acquired resistance (6). Most recently, third-generation EGFR TKIs, such as osimertinib have been introduced to overcome therapy resistance, which can evade EGFR T790M mutation by irreversibly binding to the mutated protein (7). This emphasizes the necessity of identifying patients with EGFR T790M mutation, as they may benefit from third-generation TKI therapy.
Tumor tissue biopsy has been conventionally used for tumor sampling and mutation analysis. However, it is not always possible to obtain a tissue sample when the tumor is difficult to access or a biopsy is hindered by the impaired health of the patient. Therefore, a less invasive method may be preferred to obtain tumor DNA in these cases. Cell-free circulating tumor DNA (ctDNA) regularly appears in the blood as a consequence of tumor cell apoptosis and necrosis, and has been reported to faithfully mirror the DNA content of the primary tumor of the same patient (8). Furthermore, it may better reflect the genetic heterogeneity of the tumor than a tissue biopsy (9). Hence, obtaining ctDNA from peripheral blood plasma emerged as a promising alternative to invasive tissue biopsies. Despite this fact, there are various factors which make detection of ctDNA difficult: the heavily fragmented nature and short half-life of ctDNA, low and widely variable plasma concentration levels, and the presence of background normal plasma DNA (10). This emphasizes the importance of employing a highly sensitive, reliable and accurate detection method. There are multiple diagnostic assays developed to assess EGFR status in blood plasma samples, including a number of PCR based systems, mass spectrometry, and next-generation sequencing (11). In this study, we summarized our results with the Amoydx Super-ARMS EGFR Mutation Detection Kit (Amoy Diagnostics Co., Ltd., Xiamen, China) test and we also compared the performance of the AmoyDx and the cobas EGFR Mutation test v2 (cobasv2; Roche Molecular Systems, Pleasanton, CA, United States) in peripheral plasma samples of NSCLC patients.
Material and Methods
Patients and Samples
Altogether 401 samples of 242 NSCLC patients, previously treated with first- or second-generation EGFR TKI therapy have been analyzed in the Molecular Pathology Laboratory of the National Institute of Oncology between 2019 and 2021. The number of cases in different sample types was as follows: 3 pleural effusions, 5 cell blocks, 6 cytology smears, 6 resection specimens, 17 lung biopsies and 364 blood samples. All the cytology and tissue samples contained viable tumor cells. The average number of blood samples per patient was 1.65; the maximum number was 6 blood samples per patient. Blood specimens and pleural effusions were collected and stored either in K2EDTA S-Monovette tubes (Sarstedt, Nümbrecht, Germany) at 4°C or in Cell-Free DNA BCT Streck tubes (Streck, La Vista, NE, United States) at room temperature, depending on the proximity of the sampling site. In the first case, liquid biopsies were processed within 2 h, while in the case of Streck tubes the time interval did not exceed 72 h.
The study was reviewed and approved by ETT-TUKEB, Health and Scientific Committee of Ministry of Human Resources of Hungary. Number of permission: IV/1792-4/2021/EKU.
DNA Extraction
For an optimal yield and purity of ctDNA, plasma separation was performed in a two-step centrifugation protocol with a first centrifugation step of 3000×g for 10 min and a second step of 12,000×g for 10 min (12, 13). The ctDNA isolation started immediately after the plasma separation or after a maximum of 3-day storage at −80°C. Plasma samples between 4 and 6 ml were processed with the cobas ctDNA Sample Preparation Kit (Roche) following the manufacturer’s instructions. For preparation of pleural effusion samples, 9–17 ml of the samples were centrifuged at 1700×g for 10 min to remove cells and debris. The supernatant was used for the extraction of ctDNA with the same isolation protocol as for the plasma samples. For DNA extraction from small biopsies and cytological specimens with low tumor cell contents, we preferred the ReliaPrep DNA Clean-Up and Concentration System kit (Promega, Madison, WI, United States) to achieve a highly concentrated DNA yield. As this system contains only purifying reagents, the deparaffinization and lysis steps were performed with the corresponding reagents, according to the Maxwell RSC DNA FFPE protocol (Promega). The same kit was also used for formalin fixed paraffin embedded (FFPE) resection specimens. In each case, the tumor area was macro-dissected and one to three 5-µm sections of an FFPE specimen were processed. The concentration of the DNA samples was measured by the Qubit 4 fluorometer with the Qubit™ 1X dsDNA HS (High Sensitivity) Assay Kit (Thermo Fisher Scientific, Waltham, MA, United States) and stored at 4°C until further usage. For resection specimens, Promega, ReliaPrep DNA Clean-up and Concentration System kit was used.
T790M Mutation Detection Methods
EGFR mutation detection was performed with the cobas EGFR Mutation test v2 on cobas z480 analyzer (Roche) and with the Super-ARMS EGFR Mutation Detection Kit (AmoyDx) on LightCycler 480 II instrument (Roche). All kits and devices were used as recommended by the manufacturer’s protocol. In the majority of cases, due to limited amount of DNA, only the AmoyDx kit was used as a standard method. When sufficient amount of ctDNA was available, we performed the test with both methods to compare the performances of the two cross-platform technologies.
Statistical Analysis
McNemar’s test for paired proportions was used to calculate the differences of the concordance rates of the different methods. The correlation of DNA concentration and T790M mutation detection rate was calculated with the point-biserial correlation method. Significance was calculated with two-tailed t-tests or chi-square tests.
Results
T790M Mutation Detection Results With AmoyDx Test
Four plasma samples were not suitable for ctDNA analysis due to hemolysis. The result of 17 plasma samples and one resection specimen was invalid. The average DNA concentration according to the sample type is shown in Table 1. There were 9 cases where the ctDNA concentration was higher than 10 ng/μl.

TABLE 1. Average DNA concentration, number of samples and proportion of patients showing T790M mutation according to sample type.
We also assessed if the activating EGFR mutation of the corresponding primary tumors, previously analyzed with cobas EGFR Mutation test v2, could be detected in the sample. With the AmoyDx kit, we detected the same activating EGFR mutation which was seen in the primary tumors in 181 of 397 samples (45.6%). Eighty-seven of these 181 samples (48.1%) contained T790M mutation. Out of these 87 cases, T790M mutation was detected with the AmoyDx kit in the first blood samples of the patients in 50 cases and in a subsequent second blood sample in a further 32 cases. In 4 cases, only the third blood sample was T790M positive and we had only 1 case when only the fourth blood sample showed a positive result. Further repetition of the sampling did not improve the detection rate, as we did not detect any T790M mutation after the fourth sample, when the previous samples were negative.
Considering the sample type, T790M mutation was detected at 70.3% of the patients if non-plasma samples were used, while it was detected at only 29.8% of the patients if only plasma samples were analyzed. Although the number of non-plasma samples was relatively low, the difference was significant (p < 0.0001) (Table 2). Theoretically, the lower T790M detection rate in the plasma samples could be explained by the absence of circulating tumor DNA in the sample. Nevertheless, when we included only those samples in the analysis, where the primary EGFR mutation was also identified, hence confirming the presence of ctDNA in the sample, the difference remained significant (p = 0.002444) (Table 3).
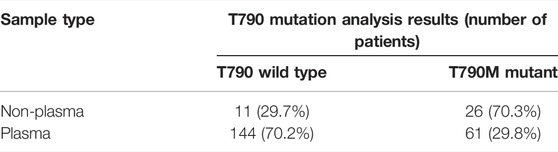
TABLE 2. Results of T790 mutation analysis of the samples of all the patients, according to the sample type (chi-square p < 0.0001).
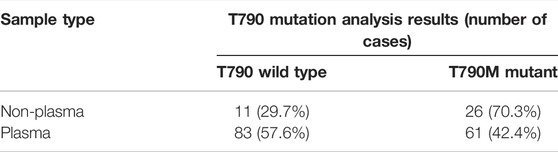
TABLE 3. Results of T790 mutation analysis when the primary activating EGFR mutation was also detected in the sample, according to the sample type (chi-square p = 0.00244).
We also examined whether the T790M mutation detection rate of the AmoyDx kit correlated with the DNA concentration. Figure 1 shows the T790M mutation frequency in relation to the DNA concentration. There was no significant correlation between the DNA concentration and the detection rate (p = 0.339), and the proportion of positive cases did not depend on the DNA concentration.
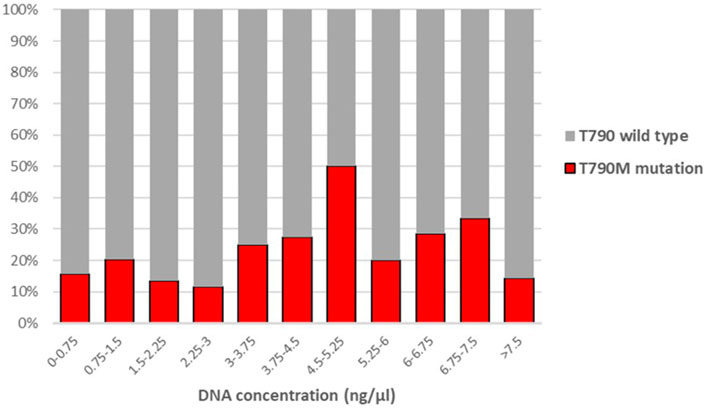
FIGURE 1. Correlation between the proportion of T790M positive cases and DNA concentration. (Point-biserial correlation p = 0.339).
The type of the primary EGFR mutations in relation to the presence of T790M mutation is shown in Table 4. T790M mutation was most frequent in exon 19 del and exon 21 mutant cases. Frequency of T790M mutation was roughly similar in EGFR exon 19 del and exon 21 mutant groups, 49 of 94 (52.1%) and 36 of 75 (48%), respectively.
Comparison of the results of cobas EGFR mutation test v2 and AmoyDx Super-ARMS test
Eighty-six plasma samples had sufficient amount of DNA for analysis with both methods. The same amount of DNA was used for comparison. These cases were also used to assess the concordance rate between the plasma samples and the previously obtained tissue samples from the same patient.
In 73 plasma samples, 85% of all cases, we obtained the same result with both tests. With the AmoyDx Super-ARMS test, 43 cases showed the same activating EGFR mutation, which was detected previously in the primary tumor (50.5%) and 20 out of these 43 EGFR mutant cases also showed T790M positivity. With cobas test, 40 cases (46%) showed the same activating EGFR mutation, which was previously detected in the primary tumor and T790M mutation was present in 14 out of these cases. Overall, 20/86 samples (23%) tested with the AmoyDx kit were T790M mutation positive vs. 14/86 (16%) with the cobas kit. The higher T790M mutation detection rate of the AmoyDx kit compared to the cobas kit was statistically significant (p = 0.014). In six samples, the T790M mutation was only detectable with the AmoyDx kit. The concordance rate of EGFR activating mutation detection between the two methods was 89%, while this was 93% for T790M mutation detection.
Seventy-one plasma samples analyzed by both AmoyDx and cobas tests had a previous tissue sample with an available EGFR mutation analysis result. Among these cases, four samples had an EGFR activating mutation detected only by the AmoyDx test, while cobas test showed a wild-type result in these cases. In one case, the same double mutation (exon 18 G719x, exon 20 S768I) was detected in the primary tumor and with AmoyDx test in the plasma sample, but the cobas test detected only the exon 20 S768I mutation. In one case, where the primary lung tumor showed exon 21 L858R mutation, the same mutation was detected with cobas test in the ctDNA, but the AmoyDx test showed an exon 20 ins. We interpreted the AmoyDx result as being false positive, since the primary tissue sample analysis showed an exon 21 L858R mutation only, although this could also be explained by tumor heterogeneity. In addition, in one case, we detected the same EGFR exon 19 del mutation with cobas test as in the primary tumor sample, but the AmoyDx gave a wild-type result. The concordance rate between the primary tissue samples and the ctDNA testing platforms is shown in Table 5. Although the AmoyDx test showed a slightly better performance in detecting the primary activating EGFR mutation in case of exon 19 del, exon 18 mut and exon 21 mut, the difference between the two platforms was not statistically significant (p = 0.563, p = 0.317, p = 0.157, respectively). The overall concordance rate with the tissue sample result was 86% for the cobas and 88% for the AmoyDx kit, the difference of which was again not statistically significant (p = 0.667).
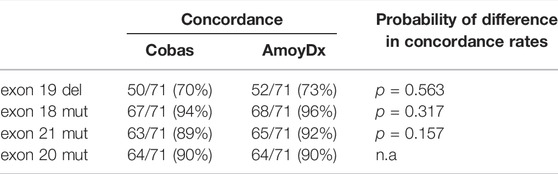
TABLE 5. Concordance between the primary tissue samples and the plasma ctDNA results for EGFR activating mutations.
Discussion
While T790M mutation occurs in less than 5% of untreated EGFR mutant lung adenocarcinomas, about 50%–70% of the EGFR mutated tumors develop T790M mutation as an acquired resistance if treated with first-generation TKIs (14). As a minimally invasive test, assessment of ctDNA in plasma samples is a valid and cost-effective alternative of tissue biopsies and identifies a large proportion of EGFR T790M mutations responsible for therapy resistance. In this study, we presented the results of EGFR T790M mutation testing in our laboratory over a 3-year period. Our results are in accordance with the literature data: as we detected T790M mutation in 48.1% of the samples, where the primary EGFR mutation could also be detected, confirming the presence of ctDNA in the sample (15). The AmoyDx test routinely used by us showed a higher detection rate of T790M mutation compared to the cobas test, and the difference was significant (p = 0.014). The concordance rate between the two tests was 93% in detecting T790M mutation and 89% in detecting the activating EGFR mutation. Compared to the previous tissue samples of the patients, the AmoyDx kit had a higher concordance rate than the cobas test kit in detecting activating EGFR mutations (86% vs. 88%), but this difference was not significant (p = 0.667). Overall, the detection rate of the AmoyDx test was slightly better than of the cobas test in our hands. However, cobas test is a very simple automated detection method with one of the widest EGFR mutation coverage among PCR based methods (42 mutations of exon 18, 19, 20, 21). Nevertheless, it was already shown previously that its sensitivity is inferior compared to ARMS techniques (allele specific polymerase chain reaction), like the AmoyDx test which also covers 42 mutations of exon 18, 19, 20 and 21, or digital PCR methods (16).
Our data suggest that plasma testing is useful in patients with EGFR T790M resistance mutations where continuous monitoring is recommended, especially if a tissue biopsy is not available. According to recent studies, in about 40% of relapsed cases it is not possible to obtain tumor tissue samples suitable for molecular analysis (17). In our hands with the AmoyDx test, T790M mutation frequency was 42.4% in those plasma samples where the activating EGFR mutation was detected. At the same time, we found that the detection of T790M mutation was not dependent on ctDNA concentration of the plasma samples. Furthermore, when multiple blood samples of a patient were sequentially taken at different points in time, the detection rate did not improve considerably after two repeated blood samples.
Although the number of non-plasma samples was very small in our study, comprising only 9.2% of the samples, the detection rate of T790M mutation was much higher than in non-plasma samples. This is in keeping with others studies on T790M testing. Tissue biopsy is still considered the gold standard for EGFR mutation analysis and up to 30% of the negative plasma samples for T790M mutation can have a positive result in a subsequent tissue biopsy (18). According to the results of Pereira et al, re-biopsy increased the detection rate of T790M mutation with 17% (19). The most probable cause of the higher detection rate of T790M in tissue samples is a higher tumor DNA content and a higher mutant allele frequency. Consequently, ctDNA mutational analysis could potentially be used as a triage test, where patients with a negative sample could undergo a further tissue biopsy. Recently, it has been demonstrated that using ctDNA as a triage test results in a superior T790M detection rate than tissue biopsy alone (20).
Re-biopsy rate can be considerably high in some practices. According to a recent Japanese publication on 120 patients with acquired resistance to EGFR-TKIs, re-biopsy was performed on 109 patients, with an implementation rate of 90.8%. However, re-biopsy is still very rare in our practice compared to the literature data. Although liquid biopsy alone is still less costly than tissue biopsy, considering its costs and effects directly relating to testing (20), re-biopsy should be considered more frequently in our practice in case of disease progression after first- and second-line EGFR TKI therapy.
Data Availability Statement
The raw data supporting the conclusion of this article will be made available by the authors, without undue reservation.
Ethics Statement
The studies involving human participants were reviewed and approved by the ETT-TUKEB, Health and Scientific Committee of Ministry of Human Resources of Hungary. Number of permission: IV/1792-4/2021/EKU. Written informed consent for participation was not required for this study in accordance with the national legislation and the institutional requirements.
Author Contributions
ET, EC, NB, and LB-F designed the study, EB and AK performed the detection of T790M mutation, NB, VS, and KB took part in the collection of patients. ET, EC, KB, and ZM wrote the manuscript.
Funding
This work was supported by the Hungarian National Laboratory (under the National Tumor Biology Laboratory project (NLP-17)) and “Befektetés a jövőbe 2020-1.1.6-JÖVŐ-2021-0008. Számú pályázat”.
Conflict of Interest
The authors declare that the research was conducted in the absence of any commercial or financial relationships that could be construed as a potential conflict of interest.
References
1. Bogos, K, Kiss, Z, Galffy, G, Tamasi, L, Ostoros, G, Muller, V, et al. Lung Cancer in Hungary. J Thorac Oncol (2020) 15(5):692–9. doi:10.1016/j.jtho.2019.11.001
2. Graham, RP, Treece, AL, Lindeman, NI, Vasalos, P, Shan, M, Jennings, LJ, et al. Worldwide Frequency of Commonly Detected EGFR Mutations. Arch Pathol Lab Med (2018) 142(2):163–7. doi:10.5858/arpa.2016-0579-CP
3. Foster, SA, Whalen, DM, Ozen, A, Wongchenko, MJ, Yin, J, Yen, I, et al. Activation Mechanism of Oncogenic Deletion Mutations in BRAF, EGFR, and HER2. Cancer Cell (2016) 29(4):477–93. doi:10.1016/j.ccell.2016.02.010
4. Morgillo, F, Della Corte, CM, Fasano, M, and Ciardiello, F. Mechanisms of Resistance to EGFR-Targeted Drugs: Lung Cancer. ESMO Open (2016) 1(3):e000060. doi:10.1136/esmoopen-2016-000060
5. Tumbrink, HL, Heimsoeth, A, and Sos, ML. The Next Tier of EGFR Resistance Mutations in Lung Cancer. Oncogene (2021) 40(1):1–11. doi:10.1038/s41388-020-01510-w
6. Yun, CH, Mengwasser, KE, Toms, AV, Woo, MS, Greulich, H, Wong, KK, et al. The T790M Mutation in EGFR Kinase Causes Drug Resistance by Increasing the Affinity for ATP. Proc Natl Acad Sci U S A (2008) 105(6):2070–5. doi:10.1073/pnas.0709662105
7. Walter, AO, Sjin, RT, Haringsma, HJ, Ohashi, K, Sun, J, Lee, K, et al. Discovery of a Mutant-Selective Covalent Inhibitor of EGFR that Overcomes T790M-Mediated Resistance in NSCLC. Cancer Discov (2013) 3(12):1404–15. doi:10.1158/2159-8290.CD-13-0314
8. Diaz, LA, and Bardelli, A. Liquid Biopsies: Genotyping Circulating Tumor DNA. J Clin Oncol (2014) 32(6):579–86. doi:10.1200/JCO.2012.45.2011
9. Fenizia, F, De Luca, A, Pasquale, R, Sacco, A, Forgione, L, Lambiase, M, et al. EGFR Mutations in Lung Cancer: from Tissue Testing to Liquid Biopsy. Future Oncol (2015) 11(11):1611–23. doi:10.2217/fon.15.23
10. Wang, M, Huang, X, Li, X, Guo, Q, Xu, W, Zhao, M, et al. Performance Comparison of Commercial Kits for Isolating and Detecting Circulating Tumor DNA. Scand J Clin Lab Invest (2021) 81(4):276–81. doi:10.1080/00365513.2020.1821394
11. Ho, HL, Huang, CC, Ku, WH, Ho, CL, Lin, CH, Yu, SL, et al. Liquid Biopsy for Detection of EGFR T790M Mutation in Nonsmall Cell Lung Cancer: An Experience of Proficiency Testing in Taiwan. J Chin Med Assoc (2019) 82(6):473–6. doi:10.1097/JCMA.0000000000000100
12. Maass, KK, Schad, PS, Finster, AME, Puranachot, P, Rosing, F, Wedig, T, et al. From Sampling to Sequencing: A Liquid Biopsy Pre-analytic Workflow to Maximize Multi-Layer Genomic Information from a Single Tube. Cancers (2021) 13(12):3002. doi:10.3390/cancers13123002
13. Sorber, L, Zwaenepoel, K, Jacobs, J, De Winne, K, Goethals, S, Reclusa, P, et al. Circulating Cell-free DNA and RNA Analysis as Liquid Biopsy: Optimal Centrifugation Protocol. Cancers (2019) 11(4):E458. doi:10.3390/cancers11040458
14. Oxnard, GR, Thress, KS, Alden, RS, Lawrance, R, Paweletz, CP, Cantarini, M, et al. Association between Plasma Genotyping and Outcomes of Treatment with Osimertinib (AZD9291) in Advanced Non-small-cell Lung Cancer. J Clin Oncol (2016) 34(28):3375–82. doi:10.1200/JCO.2016.66.7162
15. Jenkins, S, Yang, JC, Ramalingam, SS, Yu, K, Patel, S, Weston, S, et al. Plasma ctDNA Analysis for Detection of the EGFR T790M Mutation in Patients with Advanced Non-small Cell Lung Cancer. J Thorac Oncol (2017) 12(7):1061–70. doi:10.1016/j.jtho.2017.04.003
16. Li, X, and Zhou, C. Comparison of Cross-Platform Technologies for EGFR T790M Testing in Patients with Non-small Cell Lung Cancer. Oncotarget (2017) 8(59):100801–18. doi:10.18632/oncotarget.19007
17. Chouaid, C, Dujon, C, Do, P, Monnet, I, Madroszyk, A, Le Caer, H, et al. Feasibility and Clinical Impact of Re-biopsy in Advanced Non Small-Cell Lung Cancer: a Prospective Multicenter Study in a Real-World Setting (GFPC Study 12-01). Lung Cancer (2014) 86(2):170–3. doi:10.1016/j.lungcan.2014.08.016
18. Stockley, T, Souza, CA, Cheema, PK, Melosky, B, Kamel-Reid, S, Tsao, MS, et al. Evidence-based Best Practices for EGFR T790M Testing in Lung Cancer in Canada. Curr Oncol (2018) 25(2):163–9. doi:10.3747/co.25.4044
19. Pereira, I, Gaspar, C, Pina, M, Azevedo, I, and Rodrigues, A. Real-World T790M Mutation Frequency and Impact of Rebiopsy in Patients with EGFR-Mutated Advanced Non-small Cell Lung Cancer. Cureus (2020) 12(12):e12128. doi:10.7759/cureus.12128
Keywords: liquid biopsy, NSCLC, ctDNA, EGFR T790M mutation, AmoyDx super-ARMS EGFR mutation detection kit, cobas EGFR test v2
Citation: Bencze E, Bogos K, Kohánka A, Báthory-Fülöp L, Sárosi V, Csernák E, Bittner N, Melegh Z and Tóth E (2022) EGFR T790M Mutation Detection in Patients With Non-Small Cell Lung Cancer After First Line EGFR TKI Therapy: Summary of Results in a Three-Year Period and a Comparison of Commercially Available Detection Kits. Pathol. Oncol. Res. 28:1610607. doi: 10.3389/pore.2022.1610607
Received: 19 May 2022; Accepted: 22 September 2022;
Published: 05 October 2022.
Edited by:
József Tímár, Semmelweis University, HungaryCopyright © 2022 Bencze, Bogos, Kohánka, Báthory-Fülöp, Sárosi, Csernák, Bittner, Melegh and Tóth. This is an open-access article distributed under the terms of the Creative Commons Attribution License (CC BY). The use, distribution or reproduction in other forums is permitted, provided the original author(s) and the copyright owner(s) are credited and that the original publication in this journal is cited, in accordance with accepted academic practice. No use, distribution or reproduction is permitted which does not comply with these terms.
*Correspondence: Erika Tóth, ZHIudG90aC5lcmlrYUBvbmNvbC5odQ==
†These authors have contributed equally to this work and share first authorship