- 1Institute of Pathology and Molecular Genetics, University Hospital Ostrava, Ostrava, Czechia
- 2Department of Clinical and Molecular Pathology, Faculty of Medicine and Dentistry, Palacký University Olomouc, Olomouc, Czechia
- 3Department of Immunology, Faculty of Medicine and Dentistry, Palacký University Olomouc, Olomouc, Czechia
Colorectal cancer (CRC) is one of the most common cancers worldwide. The patient’s prognosis largely depends on the tumor stage at diagnosis. The pathological TNM Classification of Malignant Tumors (pTNM) staging of surgically resected cancers represents the main prognostic factor and guidance for decision-making in CRC patients. However, this approach alone is insufficient as a prognostic predictor because clinical outcomes in patients at the same histological tumor stage can still differ. Recently, significant progress in the treatment of CRC has been made due to improvements in both chemotherapy and surgical management. Immunotherapy-based approaches are one of the most rapidly developing areas of tumor therapy. This review summarizes the current knowledge about the tumor microenvironment (TME), immune response and its interactions with CRC development, immunotherapy and prognosis.
Introduction
Uncontrollable proliferation and metastasis of cancer cells within major organs, such as the liver and lung, constitute the leading causes of death in CRC patients (1). Treatment options for CRC are complex, but it must be emphasized that only some patients can undergo radical treatment of metastases in the sense of resection with curative intent. The treatment is usually based on combined chemotherapy targeting primarily the vascular endothelial growth factor receptor (VEGFR) or the epidermal growth factor (epidermal growth factor receptor–EGFR) signaling pathways (2). Unfortunately, not all patients can be treated by these selective drugs, especially EGFR inhibitors. Modern immunotherapy methods significantly expand the possibilities of treatment for patients suffering from this serious disease. This review summarizes the current knowledge about the tumor TME and the immune processes involved in the development, growth and prognosis of CRC.
Methods
We searched for keywords “immunotherapy colorectal cancer”, “tumor infiltrating lymphocytes colorectal cancer” and “PD-L1 colorectal cancer” in the database PubMed, which gave us a total of 794 studies. After discarding duplicates, we obtained 320 studies that were scanned. We excluded 240 studies unrelated to the topic, thus acquiring 65 studies that were used as a basis for this review. Further 44 papers were identified by cross-referencing.
Colorectal Cancer Tumorigenesis
Three major pathways of CRC tumorigenesis have been thoroughly described: chromosomal instability (CIN), microsatellite instability (MSI), and the CpG island methylator phenotype (CIMP) pathway (3). The tumor mutation burden (TMB) is an emerging biomarker in multiple tumor types. Highly mutated tumors are thought to harbor an increased neoantigen burden, making them immunogenic and responsive to immunotherapy (4). CIN is the most common type of genetic instability, present in 70%–85% of CRC, impacting chromosome copy number and structure (5). The CIMP pathway may contribute to silencing the tumor suppressor and DNA repair genes due to hypermethylation (6). The prevalence of CIMP varied from 21% to 27% cases of CRC across geographical regions. In some patients, both pathways may coexist; the most common combination is that of CIMP and MSI (7).
The third pathway, MSI, is a result of DNA mismatch repair deficiency (dMMR) caused by the inactivation of genes encoding proteins responsible for repairing DNA errors, mainly occurring during replication. The prognosis of tumors developing through MSI pathway is better than that of other pathways (8). Please note that the terms MSI tumors and dMMR tumors are usually used interchangeably and they will be used in this way also in this paper, with the reference to MMR preferred when speaking of the pathway and the term MSI preferred when speaking of the type of tumor. According to established guidelines, MSI is determined by a panel of microsatellite markers, commonly mononucleotides (9). Microsatellites are short repetitive DNA sequences of uniform length containing one to six bases. They are located in the non-coding regions of the genome and, less frequently, they were also identified in the regulatory or coding regions. They are highly polymorphic between subjects, but within an individual, their length is stable and uniform (10). dMMR CRCs produce a higher number of neoantigen types than MMR-proficient ones, which, consequently, leads to the greater activation of the immune system (11). MMR deficiency has been detected in approximately 15%–20% of CRC (12, 13). The sporadic microsatellite-unstable phenotype usually results from epigenetic silencing of the mismatch repair gene mutL homolog 1 (MLH1). Germline mutations of one of the broader groups of mismatch repair genes (MLH1, MSH2, MSH6, and PMS2) are typical of hereditary Lynch syndrome (14). MSI tumors are often poorly differentiated with mucinous features or a medullary growth pattern, although they can also resemble more typical CRCs with tubular or cribriform growth patterns and less pronounced mucus formation (15). Patients with MSI tumors have a more favorable prognosis than those with MS-stable tumors, which has been explained by the generally strong cytotoxic CD45RO + lymphocyte infiltration resulting from high TMB and consequently high neoantigen load (16–18). Densities of CD3+ and CD8+ cells are higher in this group of tumors, but the densities of CD4+ and transcription factor forkhead box P3 (Foxp3)+ cells were not affected by the MSI status of the tumor (15, 19).
The Role of the Immune System in the Prognosis and Treatment of Colorectal Cancer
The immune system plays an important role in cancer control and treatment response and the presence and quantity of key immune cell subtypes within the TME of CRC are known to possess prognostic potential (20). The phenotype and localization of various immune cell types (B and T cells, natural killer cells, tumor-associated macrophages and dendritic cells, myeloid-derived suppressor cells, mast cells and cancer-associated fibroblasts) in individual areas of the TME also constitute important histopathological features of prognostic value (11). Besides, immune checkpoint inhibitors, including cytotoxic T-lymphocyte-associated antigen 4 (CTLA-4) inhibitors, programmed cell death 1 (PD-1) and programmed cell death ligand 1 (PD-L1) inhibitors have been associated with significant improvement in various advanced cancers (21, 22). The role of these factors will be discussed individually below.
Role of CTLA-4 in Colorectal Cancer
CTLA-4 is considered the “leader” of immune checkpoints as it stops potentially autoreactive T cells as soon as the initial stage of naïve T-cell activation, typically in lymph nodes (21). CTLA-4 blockade was shown to inhibit tumor progression by increasing the effector T-cells activity and suppressing regulatory T cells (Treg cells) (23). The Tregs population was associated with cancer progression and metastasis. In hepatocellular carcinoma patients, for example, Han et al. identified tumor-infiltrating human CD4+CD69+ Tregs capable of suppressing CD4+ T cell response via membrane-bound transforming growth factor (TGF)-β1; the percentage of these cells significantly correlated with tumor progression (24). It is important to realize that Tregs have also the ability to inhibit the T cell-mediated immune response against tumor cells in CRC (25–27). Xu et al. reported that the function of tumor-infiltrating Tregs in a cancer nest differs from their functions in cancer stroma. In the latter, they act predominantly as inhibitors of inflammatory anti-microbial response; in a cancer nest, however, they act as suppressors of the anti-tumor response (28). The improved understanding of the detailed mechanism could help us develop new immunotherapy methods targeting CTLA-4 Tregs, ideally in specific locations.
PD-1 and PD-L1 Pathway in Colorectal Cancer
A core concept in cancer immunotherapy is that tumor cells, which would normally be recognized by T cells, have developed ways to evade the host immune system by taking advantage of peripheral tolerance and hiding from immune recognition (29). The PD-1 pathway regulates previously activated T cells, primarily in peripheral tissues (30). Takasu et al. positively correlated the activity of the PD-1 pathway with the expression of Foxp3, which is widely known to be involved in the development and function of Treg cells including CD4+CD25+ and CD8+CD25+ cells (31, 32). Several studies have confirmed that high Foxp3 expression in Treg cells allows tumor cells in various types of human carcinomas to escape from immunological surveillance and, in this way, promotes their proliferation and tumor development (33, 34). Foxp3 can be expressed also by cancer cells, which results in the secretion of immunosuppressive cytokines such as IL-10 and TGFβ into the tumor microenvironment, which might allow them to evade effector T-cell responses and, hence, to progress (35). On the other hand, Yoon et al. and Ling et al. suggest that high Foxp3 T-cell density is a favorable prognostic factor in CRCs with low cytotoxic CD8+ infiltration (36, 37). This paradox is probably caused by dense microbiological flora present in the large intestine. The colon is constantly confronted with foreign antigens, which leads to increased levels of Foxp3 T-cells even in a physiological state. A high amount of Treg cells, suppressing microbially induced inflammation, could act protectively not only by this prevention of the inflammatory response that can potentially lead to the development of cancer in the colorectal epithelium but also by preventing tumor growth; this could make it an exception to the rule of the poor prognostic role of high Foxp3+ expression (37, 38).
PD-1 and PD-L1 are crucial checkpoints of the immune system response. They provide negative feedback that inhibits T helper 1 (Th1) cytotoxic immune responses, causing T-cell exhaustion or tolerance (39). PD-L1 is constitutively expressed by T and B cells, dendritic cells, and macrophages (40), as well as by other cell types including endothelial, pancreatic, and muscle cells (41). Expression of PD-L1 either in the tumor or in the infiltrating immune cells has been verified (predominantly by immunohistochemistry) in a variety of tumors, suggesting its role as a prognostic trait and therapeutic target across multiple types of tumors. The overexpressed PD-L1 on the surface of cancer cells binds to the PD-1 on tumor-infiltrating lymphocytes (TILs), which counteracts the TCR-signaling cascade by phosphorylating SHP-2 and as a result, impairs T cell activation (42). This process is an analogy to the interaction of CD28 with the B7 molecules (CD80 or CD86) on professional antigen-presenting cells (APC); the interruption of this signaling pathway with CD28 antagonists can be typically considered a positive effect inducing suppression of the pathological immune response; in some cases, however, it can also negatively affect the organism by inducing the antigen-specific tolerance (43).
Recent reports indicated that while PD-L1 expression on CRC tumor cells was commonly associated with poor outcome or was not prognostic, lymphocytic expression of PD-L1 and PD-1 in CRC was associated with good clinical outcome, particularly in certain colon locations (44, 45). PD-1 and PD-L1 expressions are regulated by numerous factors, including inflammatory stimuli (IFN, IL-6, TNF) and oncogenic pathways at transcription, post-transcription, and post-translation (e.g., EGFR, MAPK, JAK-STAT pathway) levels (46).
Inhibition of the PD-1/PD-L1 signaling is a feasible strategy for normalizing the TME and up to now, this approach has been used in the treatment of various cancers, such as melanoma, non-small cell lung cancer, gastric cancer, renal cancer, liver cancer, urothelial cancer, lymphoma, and all microsatellite instability-high (MSI-H) cancers (47). Noh et al. hypothesized that PD-L1 overexpression in CRC tumor cells is associated with broader activation of CD8+ T cells, which leads, among other things, to enhanced local expression of supporting factors such as IFN-γ. Enhanced CD8 recognition of CRC cells could be also linked to increased or aberrant expression of normal proteins due to the increased number of nonsynonymous, somatic mutations and MSI in tumor cells (48, 49). Practically, the expression of PD-L1 is primarily attributed to tumor-associated immune cells, most prominent at the tumor-stroma interface along the invasive margin. The true expression of PD-L1 by a tumor cell is a rare event (50).
Clinicopathologically, PD-L1 positivity, as well as PD-1 density, tend to associate with proximal tumor location and poor differentiation (51), and these features are commonly seen in MSI-H CRCs. On the contrary, mismatch repair-proficient tumors have usually high budding and show a low PD-L1 and PD-1 positivity (52). Generally, the low number of PD-L1 positive tumor samples may suggest that the PD-1/PD-L1 checkpoint mechanism is not the major escape mechanism for tumor cells in CRC, which could also explain the limited impact of anti-PD-L1/PD-1 therapy in this type of cancer (53). Shen et al. suggested that the expression of PD-L1 could be utilized as an independent factor in judging the prognosis of CRC, and reported that patients with advanced cancer or lymphatic invasion were more likely to express PD-L1 (54).
According to recent resources, the expression of PD-L1 in immune cells is significantly higher in MSI CRCs when compared to non-MSI tumors (50), which is believed to be one of the reasons for the better prognosis of these tumors (55). Ganesh et al. suggest that PD-1 blocking therapy potentially benefits patients with MMR/MSI subsets of CRCs (56). The reported overall objective response rate was 36% (51). In 2017, the PD-1 inhibitor Pembrolizumab was approved by the US Food and Drug Administration for treatment of metastatic solid MSI tumors, including CRC (57). Nevertheless, it has been speculated that with disease progression and development of metastases, dMMR/MSI tumors evade host immune surveillance, which is in turn associated with a loss of the prognostic advantage. This is seen in stage IV CRCs with dMMR/MSI where no prognostic advantage was found (58). Unfortunately, as MSI tumors represent only about 15 % of CRCs, the monotherapy with immune checkpoint inhibitors has shown only limited efficacy when applied to the general CRC population (53). All these facts indicate that the function of PD-L1 is complicated and diverse. This, in turn, means that in tumor-targeted therapy, the inhibition of either of these factors (PD-L1 or PD-1) alone is likely to fail in the blocking of the full PD-1/PD-L1 pathway and, hence, although it may act well in patients at lower and not-generalized stages, it is unlikely to universally help patients at all stages of the disease.
Chronic T cell activation, which may occur in persistent infections or cancer following repeated exposures to antigens, is also associated with an increased expression of inhibitory receptors such as PD-1 and CTLA-4, which leads to T cell dysfunction compromising productive T cell responses (59, 60). Yassin et al. found in experimental mice that chronic inflammation resulting in colitis-associated cancer development leads to a massive PD-1 upregulation in intraepithelial lymphocytes. This upregulation affects certain CD4 and CD8 (CD8αβ+, CD8αα+; TCRγδ+, TCRαβ+) subsets and may contribute to the reduced protective capacity of early innate (TCRγδ+) and subsequent adaptive (TCRαβ+) T cell systems (61). In addition, alterations of fecal and mucosal microbiota with reduction of bacterial species diversity have been reported in CRC patients at different cancer stages (62, 63). It follows that the immune processes themselves may be affected not only by tumor cells but also by other events taking place in adjacent areas of the tumor and which may also (negatively or positively) affect the prognosis and course of CRC. Therefore, we should not focus solely on examining the tumor, but events taking place in its vicinity need to be studied as well.
Other Immune Processes in Colorectal Cancer Tumor Microenvironment
In addition to PD-1/PD-L1 signaling inhibition, immunotherapy relying on the combination of multiple checkpoint targets is another viable possibility for (not only) CRC treatment. Fiegle et al. showed that the dual CTLA-4 and PD-L1 blockade exerts synergistic inhibitory effects on the growth and metastasis of CRC in orthotopic mice by increasing the number of CD8+ and CD4+ T cells. This increase is associated with a Th1 response mediated by CTLA-4 inhibition and by inducing a higher number of M1 macrophages, which can mostly be ascribed to PD-L1 blockade (12). Kuwahara et al. showed that high CD4+ T-cell density was associated with a positive CRC outcome and constituted an independent prognostic factor in a multivariate model of CRC prognosis; its prognostic value even exceeded those of tumor invasion depth or positive lymph node metastasis (64). Previous studies have also reported that in the absence of modulation by CD4+ cells, specific CD8+ cells can become lethargic and cannot transform into long-lived functional effector cells (65, 66). However, several subsets of CD4+ TILs differing in their anti/protumor action were identified. One such subset, Th1 cells, activates CD8+ T cells, thus enhancing antitumor immunity (67). On the other hand, another subset, T helper 2 (Th2) cells, seem to suppress antitumor immunity via the production of immunosuppressive cytokines such as interleukin (IL)-10. A third subset, T helper 17 (Th17) cells, is reported to have both positive (facilitating antitumor immune response) and negative (increasing tumor growth by inducing tumor neoangiogenesis) effects (11). Thus, CD4+ T cells have a central role in managing and regulating the immune system against tumor cells.
During T cell activation, PD-1 is expressed on the surface of T cells as well as B cells and NK cells (68). Predominantly, however, it affects CD8+ T cells as the first line of defense against tumor cells and contributes to controlling T cell exhaustion (69). These cells, such as TILs, form the first line of the adaptive immune responses to a tumor (70). After arriving in the TME, CD8+ T cells should encounter both intrinsic (CD28, CTLA-4, PD-1/PD-L1, immunoglobulin-like transcript receptors), and extrinsic (regulatory T cells and myeloid cells) checkpoint regulators (71). High CD8+ T cell density is, therefore, another good prognostic marker in CRC, indicating an “immunologically hot” tumor microenvironment (20, 72). Reissfelder et al. highlighted the importance of TNF-α as a tissue marker of intratumoral cytotoxic T cell activity, reporting a strong correlation between the up-regulation of TNF-α expression in TILs and the total intratumoral TNF-α (73).
Also, intratumoral heterogeneity needs to be considered, in particular the differences between the tumor center and tumor margin. Kwak et al. demonstrated that the higher total number of CD3+ and CD8+ T cells in the immune infiltrate are predictive of survival. This is true for both the invasive margin and tumor center; the presence of these T cells in both these parts of the tumor further improves the prognosis (74). Miller et al. also observed an association of higher densities of PD-L1+ dendritic cells in the tumor margin with good survival (75). Dahlin et al. say that the separate measurements of T cell infiltration in the tumor margin, center, and intraepithelial compartment were closely correlated and were all positively associated with cancer-related survival (76). It follows that examining the tumor as a whole and not taking its heterogeneity into account might fail to provide us with satisfactory prognoses and results.
Some studies found that a high total lymphocyte score predicted cancer-related survival more accurately than any of the individual lymphocyte scores analyzed separately (16, 77). Ling et al. reported significantly better prognosis of patients with high levels of intraepithelial CD8+ T cells. Similarly, improved prognosis was reported in patients with low levels of intraepithelial CD8+ together with high concentration of Foxp3+ cells at the tumor invasive margin and in the centre (37). This observation was explained by the organ specific contribution of Foxp3 T cells to blocking tumor promoting inflammation stimulated by gastrointestinal bacteria, or eventually by unique cytokine profile (IL-2 and IFN-γ) or non-regulatory conventional function of at least some CRC-infiltrating Foxp3 T cells. Huang et al. also reported, compared with other subgroups, better survival outcomes in the subgroup of patients with the combination of high CD8+ TIL infiltration and high PD-L1 expression in tumor cells (78). Authors proposed that IFN-γ secreted by infiltrating cytotoxic CD8+ T cells is required for PD-L1 induction, and overexpression of PD-L1 within the tumor microenvironment acts as a negative feedback compensating for CD8+ CTLs and IFN-γ.
There are, of course, also other immune cells with specialized functions. NK cells are involved in the lysis of tumor cells that lose MHC class I expression (79). TME infiltration by NK alongside the CD8+ T cells has been also associated with improved prognosis in CRC patients (80).
B cells can modulate immune responses through various mechanisms, including inhibition of T cell responses (81). Shimabakuro-Vornhagen et al. showed that compared with the circulation of healthy individuals, the levels of highly activated and memory B cells present in the circulation of CRC patients (as well as in their TME) were elevated (82). Toor et al. showed that the total B cells levels were similar between tumor and normal tissue but in the TME, B cells expressed significantly higher levels of co-stimulatory immune checkpoints (83). This co-expression of multiple immune checkpoints can have important implications on tumor resistance to immunotherapy due to the overlap in their pathways, which may promote tumor progression (84, 85) and may be one of the reasons for the failure of immunotherapy even in patients in whom success could be otherwise.
The Role of Innate Immune Cells
The innate immune components of the microenvironment represent the first line of defense, providing rapid response to foreign factors as well as anti-tumor activities (86). Macrophages, a component of the mononuclear phagocytic system (MPS), play a crucial role in maintaining the tissue homeostasis through regulation of the innate immune as well as inflammatory responses. Through specific differentiation, macrophages can evolve into two distinct differentiation states: classically activated M1 (proinflammatory) macrophages (87), which could promote the Th1 response, absorb and kill tumor cells (88), or alternatively, evolve into activated M2 (anti-inflammatory) macrophages, which secret anti-inflammatory cytokines such as interleukin-10 (IL-10), and promote angiogenesis, tissue remodeling, injury repair, and tumor initiation and progression (89). Upon stimulation, macrophages recruit monocytes from the blood to the tumor site and contribute to changing toward tumor-associated macrophages (TAMs) (90). In the early stage of cancer, TAMs are represented mainly by the proinflammatory M1 macrophages, and they produce reactive oxygen and nitrogen species (91); at this stage, they have antitumor effect. Later, however, tumor cells use TAMs to support CRC and (through multiple signaling pathways) convert them into anti-inflammatory and cancer-promoting M2 phenotypes with tumorigenic activity (92). The role of TAMs in CRC is, therefore, highly complicated and their simple presence or absence is not a clear indicator of the patient’s prognosis and overall survival. It, however, appears that their presence in the early stages of cancer is rather a positive prognostic marker while in the later stages, it affects the prognosis negatively.
Dendritic cells (DCs) are professional antigen-presenting cells and their interrupted development allows tumor cells to evade immune recognition. To avoid immune surveillance, cancer cells may suppress DCs through multiple mechanisms, including the secretion of immunosuppressive TGF-β or IL-10 (93). It was reported that these DCs secrete increased levels of immunosuppressive IL-10 and decreased levels of immunostimulatory IL-12 and TNF-α (94) or the chemokine (C-X-C motif) ligand 1(CXCL1), which enhances tumor cell migration (95). Several studies reported that the number and functions of blood DC subsets were reduced in CRC patients, demonstrating that the range of these effects correlated with the disease stage and prognosis (94, 96). Another point of view is offered by the work of Miller et al., which demonstrated that PD-L1+ tumor-associated dendritic cells correlate with improved survival in CRC patients, which may reflect an active T cells-driven anti-tumor immune response (75). These observations are, however, not mechanistically reliably explained yet and the situation is similar to TAMs.
Mast cells (MCs) are multifunctional cells whose role is to participate in allergic and infectious events. In cancers, their presence has been described mainly at the tumor margins and at peri-vascular regions. It was associated with increased blood vessel density in the TME (97) and with poor prognosis (98). According to Zhao et al., a lower frequency of circulating MCs and their progenitors was found in patients with CRC than in healthy patients; this was especially apparent in patients with advanced disease. It turned out that this may reflect aggressive CRC course (99).
Myeloid-derived suppressor cells (MDSC) represent a heterogeneous population of granulocytes and monocytes that rapidly expand during infection, inflammation, and cancer (100). They have been reported to suppress T cell response through reactive oxygen species (ROS) inducing antigen-specific tolerance as well as through elevating inducible nitric oxide synthase (iNOS), arginase-I (ARG1) and other suppressive cytokines (101, 102). Their recruitment depends on various CRC-derived mediators including chemokines, with chemokine (C-C motif) ligand 2 (CCL2) being particularly relevant. MDSC recruited by the CCL2-CCR2 (C-C chemokine receptor type 2) signaling pathway in mice models were shown to support CRC growth (103). Their relevance for predicting patient prognosis, however, remains unknown. Innate immunity cells contribute to the protection from tumor cells proliferation particularly at early stage of tumor development. At the clinically manifested stage their protective effect is substantially limited.
It follows that most of the immune cells may actually have a dual activity—anti- and pro-tumor, depending on the signals received from the TME. These signals may support anti-tumorigenic functions or modulate immune cells into a protumorigenic phenotype. This knowledge reflects the potential and importance of the role of TME and the potential of treatment approaches targeting TME for the treatment of CRC. As an example, we can mention therapies blocking CTLA-4, PD-1/PD-L1 pathways, those limiting monocyte infiltration, reprogramming polarization of TAMs, blocking secretion of immunosuppressive TGF-β or IL-10 by cancer cells, or inhibiting the activity of MDSCs.
Tumor Microenvironment and Immune Response to Colorectal Cancer
Various mechanisms have been proposed to explain how the TME contributes to tumor progression, tumor invasion, and metastasis. For instance, the following mechanisms were proposed: 1) impacting the proliferation and survival of cancer cells; 2) increasing their stem-like properties and favoring epithelial-to-mesenchymal transition (EMT); (104–106), 3) rewiring the tumor metabolism (107) and/or 4) stimulating metastatic dissemination (108). EMT was shown to be a pivotal driver of fibrosis development in embryonic tissues, wound healing, tumorigenesis, and metastasis (109). It is a dynamic process involving not only pure epithelial or mesenchymal cells but also “hybrid” cells (110). Previous studies have suggested EMT activation in a number of malignant tumor cells, resulting in the loss of typical characteristics of epithelial cells (cell-cell junctions and apical basal polarity), and acquiring properties of mesenchymal cells, which in turn promotes migration and invasion-metastasis cascade (109, 111). Cancer cells with mesenchymal phenotypes are also less susceptible to cytotoxic lymphocyte-mediated lysis and NK cell attacks (112). EMT is mediated by immune cells in the TME secreting cytokines, inflammatory factors, and chemokines. Also, cancer cells can crosstalk with immune cells to induce cell plasticity and release immunosuppressive substances, thus creating an immunosuppressive microenvironment that promotes invasion and metastasis (113, 114).
Cancer-associated fibroblasts (CAFs), non-neoplastic cells present in the tumor, are a major contributor to EMT. CAFs produce desmoplastic reaction (DR), commonly observed in invasive CRC. Desmoplasia is the rearrangement of organized, anisotropic extracellular matrix fibers in a pathological microenvironment associated with metastasis or poor patient survival (115). Immature desmoplasia is characteristic of tumors with keloid-like collagen or myxoid stroma, which have been associated with poor survival in CRC patients (116). Periostin (POSTN), as a product of CAFs, could be another key molecule contributing to the malignant potential of CRC by modifying the desmoplastic environment (117). CAFs in tumor tissue also antagonize the T-cell antitumor activity and negatively contribute to patients’ prognosis (118). Monitoring of desmoplastic reactions, therefore, appears to be a potentially promising prognostic parameter.
Immunoscoring
Evaluation of the amount and type of TIL seems to be a suitable complement to the standard TNM classification, especially when deciding on adjuvant therapy. The immunoscore (IS) methodology quantifies and detects various types of immune cells in tumor tissue, and, besides, determines the density of their infiltration and localization in the center and invasive margin. It offers ratings from 0 (low immune system cell infiltration in both areas) to 4 (high immune system cell infiltration in both areas). There are two advantages to this examination: 1) IS appears to be a strong prognostic factor for disease-free survival and overall survival and 2) it has biological significance (adaptive and also an innate immune response to the presence of tumor cells). It can, therefore, be also used as a tool for the management of therapy, including immunotherapy (22, 119–121). In addition, adaptive cell therapy using TILs from patients, donors, or differentiated from stem cells, is a highly promising immunotherapeutic strategy in CRC patients. These immune cells are activated and expanded in vitro (and, if need be, possibly subjected to gene modifications) before finally being administered to the patient (122). Beak and Kim obtained TILs from patients with CRC and evaluated their potential as an immunotherapeutic modality. They demonstrated that the ex vivo expanded TILs contained mostly the effector memory T cell subset and elicited anti-tumor response (123). Koelzer et al. have demonstrated CD8+ and CD45RO + memory T cell infiltration in preoperative biopsies to be reliable independent prognostic markers associated with abundant biological behavior after resection (independently of the TNM stage and postoperative therapy) (Table 1). The high level of this infiltration predicts a locally less advanced tumor along with an absence of nodal metastases and lymphovascular invasion (124).
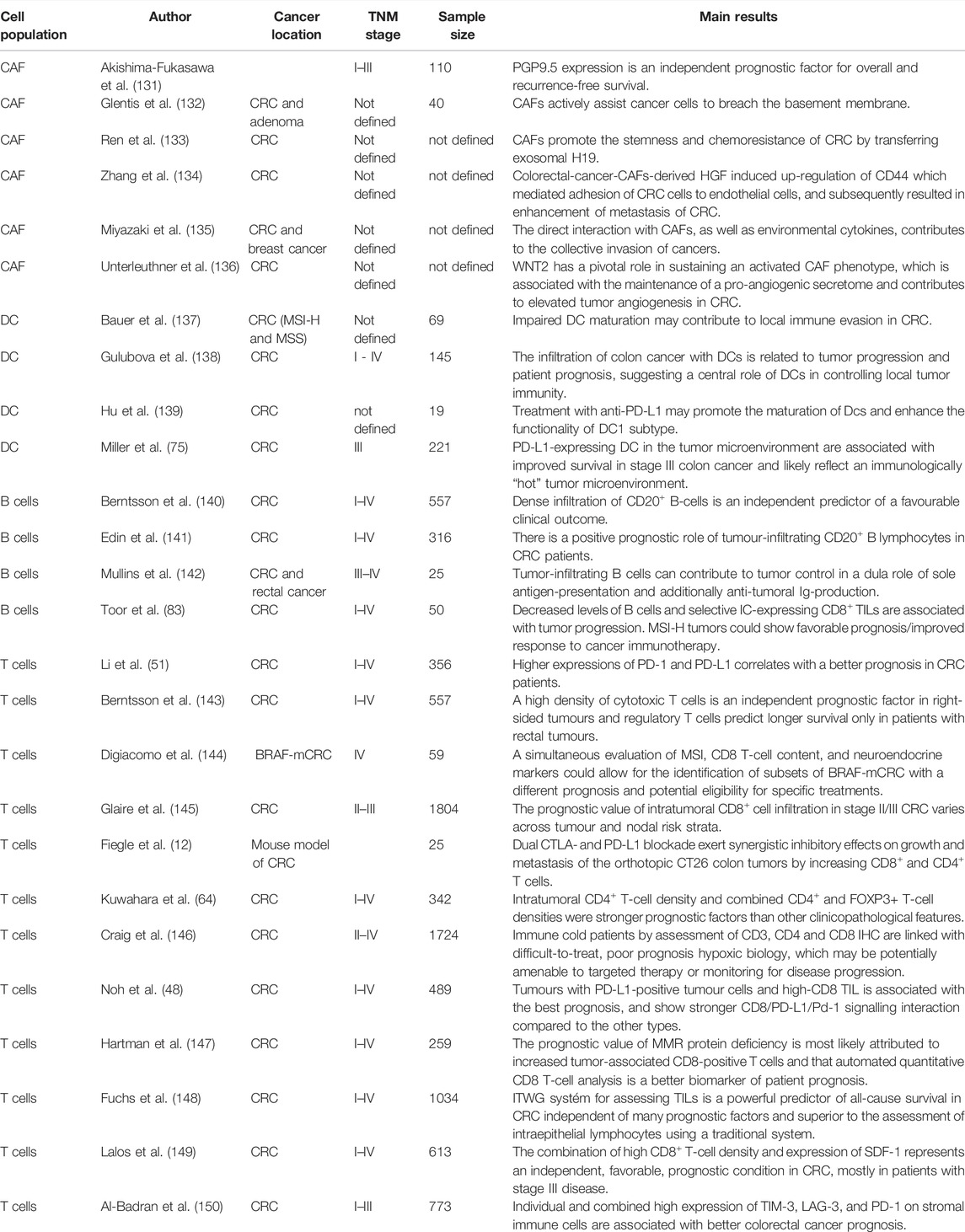
TABLE 1. Landmark studies indicating the value of different cell populations in predicting CRC prognosis.
The predictive potential of IS has been reported in several studies. For example, Morris et al. reported longer overall survival in patients with TILs who received adjuvant chemotherapy based on 5-fluorouracil (125). Similarly, the high density of CD3+, CD8+ and granzyme B+ lymphocytes in the invasive margin of hepatic CRC metastases was predictive of prolonged disease-free survival in patients treated with conventional chemotherapy or chemotherapy in combination with cetuximab or bevacizumab (126). However, more studies and more patients will be needed for validating and improving the IS sufficiently to be used in routine clinical practice.
Consensus Molecular Subtypes
The analyses of the effects of the immune infiltrate in specific subgroups of patients and their clinical outcome led to the development of a new classification system based on the consensus between different classification systems proposed by various research groups. Thus, four “consensus molecular subtypes” (CMSs) of CRC were defined (8): CMS1 is characterized by MSI, mutations in CIMP and BRAF pathways, a diffuse immune infiltrate, and strong activation of immune evasion pathways. CMS2 tumors show high chromosomal instability and activation of Wnt and MYC pathways. CMS3 tumors exhibit frequent KRAS mutations and disrupted metabolic pathways, and CMS4 tumors are characterized by high expression of mesenchymal genes, stromal infiltration, angiogenesis, and TGF-β activation. CMS1 and CMS4 are tumors characterized by strong immune infiltration while, on the contrary, CMS2 and CMS3 are tumors without immune activation. Their prognoses also differ, with CMS4 tumors displaying worse overall and relapse-free survivals (8). This classification provides an interesting opportunity to explore the heterogeneity of CRC. Understanding CMS is a crucial step towards personalized medicine as it could optimize the management of individual patients. For example, it will allow defining the best first-line chemotherapy regimen (poor efficiency of oxaliplatin in CMS1 and CMS4) or application of immunotherapy for metastatic CRC in CMS4 patients (127).
The biological and prognostic associations of CMS in the context of metastatic tumor heterogeneity is slightly more complicated. Eide et al. performed exploratory analyses of a dataset of 317 primary tumor samples and 295 liver metastasis samples. Almost 90% of metastases belonged to CMS2 or CMS4 subtypes, which is in line with the aforementioned poorer prognosis of these two subtypes (128). These results were further corroborated by Fontana et al. and Kamal et al. (129, 130). The difference is caused by a combination of biological intrinsic and extrinsic factors, including the reaction to treatment; for example, metastatic tumors after chemotherapy have been shown to tend towards CMS4 classification, even if the primary tumor was another subtype with originally better prognosis (such as CMS3) (130).
However, it must be considered that even within CMS groups, there is high inter- and intratumor heterogeneity, which limits universal application of the CMS classification in the clinical practice as a predictive prognostic marker. In view of this, further subclassification based, for example, on detailed gene expression profiling methods like single-cell RNA sequencing and analyses might refine this classification system and improve its clinical usability.
Conclusion
This review shows us that despite the large amount of available information and relatively detailed knowledge of the immune mechanisms that take place inside the tumors and in their vicinity, many factors and variables remain unclear, which ultimately determine success of proposed therapy. The immune contexture of each CRC is highly relevant for the design and allocation of therapeutic approaches that depend on anti-tumoral immune responses and include conventional therapeutic options (chemo- and radiotherapy) as well as immunotherapy. PD-L1+ positive tumor cells and CD8+ TIL are, therefore, key prognostic biomarkers for locally advanced CRC patients treated with neoadjuvant chemoradiotherapy. Upregulation of immune response with immunotherapy has been successful in a subset of patients with dMMR/MSI-H CRC patients. Choosing the right combination of drugs and the right therapeutic strategy for each individual patient including those with metastasized CRC is the key to successful therapy. These combinations and individualization will be crucial aspects of a future clinical trial also at our department. Future directions in the study of immunotherapy in CRC will include identifying improved biomarkers in patients with pMMR/MSS, and identifying novel immunotherapies with improved efficacy, such as specific treatments targeting innate immune cells supporting tumor growth.
Data Availability Statement
The original contributions presented in the study are included in the article/supplementary material, further inquiries can be directed to the corresponding author.
Author Contributions
MW and JS did the literature research and selected relevant studies. MW summarized data from these studies and wrote the manuscript. MR made the major revision of the text. All authors contributed to manuscript revision, read, and approved the submitted version.
Funding
This work was supported by MH CZ–DRO-FNOs/2016 and NU21-03-00372.
Conflict of Interest
The authors declare that the research was conducted in the absence of any commercial or financial relationships that could be construed as a potential conflict of interest.
References
1. Creasy, JM, Sadot, E, Koerkamp, BG, Chou, JF, Gonen, M, Kemeny, NE, et al. Actual 10-year Survival after Hepatic Resection of Colorectal Liver Metastases: What Factors Preclude Cure? Surgery (2018) 163:1238–44. doi:10.1016/j.surg.2018.01.004
2. Tomášek, J, and Kiss, I. Immunotherapy of Colorectal and Anal Cancer. Klin Onkol (2017) 30(3):3562–5. doi:10.14735/amko20173S62
3. Grady, WM, and Pritchard, CC. Molecular Alterations and Biomarkers in Colorectal Cancer. Toxicol Pathol (2014) 42:124–39. doi:10.1177/0192623313505155
4. McGranahan, N, Furness, AJS, Rosenthal, R, Ramskov, S, Lyngaa, R, Saini, SK, et al. Clonal Neoantigens Elicit T Cell Immunoreactivity and Sensitivity to Immune Checkpoint Blockade. Science (2016) 351(6280):1463–9. doi:10.1126/science.aaf1490
5. Tsang, AHF, Cheng, KH, Wong, ASP, Ng, SS, Ma, BB, Chan, CML, et al. Current and Future Molecular Diagnostics in Colorectal Cancer and Colorectal Adenoma. World J Gastroenterol (2014) 20:3847–57. doi:10.3748/wjg.v20.i14.3847
6. Damilakis, E, Mavroudis, D, Sfakianaki, M, and Souglakos, J. Immunotherapy in Metastatic Colorectal Cancer: Could the Latest Developments Hold the Key to Improving Patient Survival? Cancers (2020) 12:889. doi:10.3390/cancers12040889
7. Advani, SM, Advani, PS, Brown, DW, DeSantis, SM, Korphaisarn, K, VonVille, HM, et al. Global Differences in the Prevalence of the CpG Island Methylator Phenotype of Colorectal Cancer. BMC Cancer (2019) 19:964. doi:10.1186/s12885-019-6144-9
8. Guinney, J, Dienstmann, R, Wang, X, de Reynies, A, Schlicker, A, Soneson, C, et al. The Consensus Molecular Subtypes of Colorectal Cancer. Nat Med (2015) 21:1350–6. doi:10.1038/nm.3967
9. Umar, A, Boland, CR, Terdiman, JP, Syngal, S, de la Chapelle, A, Ruschoff, J, et al. Revised Bethesda Guidelines for Hereditary Nonpolyposis Colorectal Cancer (Lynch Syndrome) and Microsatellite Instability. J Natl Cancer Inst (2004) 96:261–8. doi:10.1093/jnci/djh034
10. Llosa, NJ, Cruise, M, Tam, A, Wicks, EC, Hechenbleikner, EM, Taube, JM, et al. The Vigorous Immune Microenvironment of Microsatellite Instable colon Cancer is Balanced by Multiple Counter-inhibitory Checkpoints. Cancer Discov (2015) 5(1):43–51. doi:10.1158/2159-8290.CD-14-0863
11. Fridman, WH, Zitvogel, L, Sautes-Fridman, C, and Kroemer, G. The Immune Contexture in Cancer Prognosis and Treatment. Nat Rev Clin Oncol (2017) 14:717–34. doi:10.1038/nrclinonc.2017.101
12. Fiegle, E, Doleschel, D, Koletnik, S, Rix, A, Weiskirchen, R, Borkham-Kamphorst, E, et al. Dual CTLA-4 and PD-L1 Blockade Inhibits Tumor Growth and Liver Metastasis in a Highly Aggressive Orthotopic Mouse Model of Colon Cancer. Neoplasia (2019) 21:932–44. doi:10.1016/j.neo.2019.07.006
13. Imai, K, and Yamamoto, H. Carcinogenesis and Microsatellite Instability: The Interrelationship between Genetics and Epigenetics. Carcinogenesis (2008) 29:673–80. doi:10.1093/carcin/bgm228
14. Lynch, H, Lynch, P, Lanspa, S, Snyder, C, Lynch, J, Boland, C, et al. Review of the Lynch Syndrome: History, Molecular Genetics, Screening, Differential Diagnosis, and Medicolegal Ramifications. Clin Genet (2009) 76:1–18. doi:10.1111/j.1399-0004.2009.01230.x
15. Jenkins, MA, Hayashi, S, O´Shea, AM, Burgart, LJ, Smyrk, TC, Shimizu, D, et al. Pathology Features in Bethesda Guidelines Predict Colorectal Cancer Microsatellite Instability: A Population-Based Study. Gastroenterology (2007) 133:48–56. doi:10.1053/j.gastro.2007.04.044
16. Nosho, K, Baba, Y, Tanaka, N, Shima, K, Hayashi, M, Meyerhardt, JA, et al. Tumor-infiltrating T-Cell Subsets, Molecular Changes in Colorectal Cancer, and Prognosis: Cohort Study and Literature Review. J Pathol (2010) 12:298–306. doi:10.1002/path.2774
17. Ogino, S, Nosho, K, Irahara, N, Meyerhardt, JA, Baba, Y, Shima, K, et al. Lymphocytic Reaction to Colorectal Cancer is Associated with Longer Survival, Independent of Lymph Node Count, Microsatellite Instability, and CpG Island Methylator Phenotype. Clin Cancer Res (2009) 15:6412–20. doi:10.1158/1078-0432.CCR-09-1438
18. Fabrizio, DA, George, TJ, Dunne, RF, Frampton, G, Sun, J, Gowen, K, et al. Beyond Microsatellite Testing: Assessment of Tumor Mutational burden Identifies Subsets of Colorectal Cancer Who May Respond to Immune Checkpoint Inhibition. J Gastrointest Oncol (2018) 9(4):610–7. doi:10.21037/jgo.2018.05.06
19. Salama, P, Phillips, M, Grieu, F, Morris, M, Zeps, N, Joseph, D, et al. Tumor-infiltrating FOXP3+ T Regulatory Cells Show strong Prognostic Significance in Colorectal Cancer. J Clin Oncol (2009) 27:186–92. doi:10.1200/JCO.2008.18.7229
20. Angell, HK, Bruni, D, Barett, JC, Herbst, R, and Galon, J. The Immunoscore: Colon Cancer and beyond. Clin Cancer Res (2020) 26:332–9. doi:10.1158/1078-0432.CCR-18-1851
21. Krummel, MF, and Allison, JP. CD28 and CTLA-4 Have Opposing Effects on the Response of T Cells to Stimulation. J Exp Med (1995) 182:459–65. doi:10.1084/jem.182.2.459
22. Brahmer, JR, Tykodi, SS, Chow, LQ, Hwu, WJ, Topalian, SL, Hwu, P, et al. Safety and Activity of Anti-PD-L1 Antibody in Patients with Advanced Cancer. N Engl J Med (2012) 366(26):2455–65. doi:10.1056/NEJMoa1200694
23. Pardoll, DM. The Blockade of Immune Checkpoints in Cancer Immunotherapy. Nat Rev Cancer (2012) 4:252–64. doi:10.1038/nrc3239
24. Han, Y, Yang, Y, Chen, Z, Jiang, Z, Gu, Y, Liu, Y, et al. Human Hepatocellular Carcinoma-Infiltrating CD4+ CD69+ Foxp3- Regulatory T Cell Suppresses T Cell Response via Membrane-Bound TGF-Beta1. J Mol Med (2014) 95(5):539–50. doi:10.1007/s00109-014-1143-4
25. Svensson, H, Olofsson, V, Lundin, S, Yakkala, C, Bjorck, S, Borjesson, L, et al. Accumulation of CCR4+CTLA-4hi FOXP3+CD25hi Regulatory T Cells in colon Adenocarcinomas Correlate to Reduced Activation of Conventional T Cells. PLoS One (2012) 7:e30695. doi:10.1371/journal.pone.0030695
26. Liu, Y, Xia, T, Jin, CH, Gu, D, Yu, J, Shi, W, et al. FOXP3 and CEACAM6 Expression and T Cell Infiltration in the Occurrence and Development of colon Cancer. Oncol Lett (2016) 11:3693–701. doi:10.3892/ol.2016.4439
27. Deschoolmeester, V, Baay, M, Lardon, F, Pauwels, P, and Peeters, M. Immune Cells in Colorectal Cancer: Prognostic Relevance and Role of MSI. Cancer Microenviron (2011) 4:377–92. doi:10.1007/s12307-011-0068-5
28. Xu, W, Liu, H, Song, J, Fu, HX, Qiu, L, Zhang, BF, et al. The Appearance of Tregs in Cancer Nest is a Promising Independent Risk Factor in colon Cancer. J Cancer Res Clin Oncol (2013) 139:1845–52. doi:10.1007/s00432-013-1500-7
29. Poschke, I, Mougiakakos, D, and Kiessling, R. Camouflage and Sabotage: Tumor Escape from the Immune System. Cancer Immunol Immunother (2011) 60:1161–71. doi:10.1007/s00262-011-1012-8
30. Fife, BT, and Bluestone, JA. Control of Peripheral T-Cell Tolerance and Autoimmunity via the CTLA-4 and PD-1 Pathways. Immunol Rev (2008) 224:166–82. doi:10.1111/j.1600-065X.2008.00662.x
31. Takasu, C, Nishi, M, Yoshikawa, K, Tokunaga, T, Kashihara, H, Yoshimoto, T, et al. Impact of Sidedness of Colorectal Cancer on Tumor Immunity. PLoS ONE (2020) 15(10):e0240408. doi:10.1371/journal.pone.0240408
32. Fontenot, JD, Rasmussen, JP, Williams, LM, Dooley, JL, Farr, AG, Rudensky, AY, et al. Regulatory T Cell Lineage Specification by the Forkhead Transcription Factor Foxp3. Immunity (2005) 22:329–41. doi:10.1016/j.immuni.2005.01.016
33. Li, W, Wang, L, Katoh, H, Liu, R, Zheng, P, Liu, Y, et al. Identification of a Tumor Suppressor Relay between the FOXP3 and the Hippo Pathways in Breast and Prostate Cancers. Cancer Res (2011) 71:2162–71. doi:10.1158/0008-5472.CAN-10-3268
34. Zuo, T, Liu, R, Zhang, H, Chang, X, Liu, Y, Wang, L, et al. FOXP3 is a Novel Transcriptional Repressor for the Breast Cancer Oncogene SKP2. J Clin Invest (2007) 117:3765–73. doi:10.1172/JCI32538
35. Kim, M, Grimmig, T, Grimm, M, Lazariotou, M, Meier, E, Rosenwald, A, et al. Expression of Foxp3 in Colorectal Cancer but Not in Treg Cells Correlates with Disease Progression in Patients with Colorectal Cancer. PLoS One (2013) 8(1):e53630. doi:10.1371/journal.pone.0053630
36. Yoon, HH, Orrock, JM, Foster, NR, Sargent, DJ, Smyrk, TC, Sinicrope, FA, et al. Prognostic Impact of FoxP3+ Regulatory T Cells in Relation to CD8+ T Lymphocyte Density in Human Colon Carcinomas. PLoS ONE (2012) 7(8):e42274. doi:10.1371/journal.pone.0042274
37. Ling, A, Edin, S, Wikberg, ML, Öberg, Å, and Palmqvist, R. The Intratumoural Subsite and Relation of CD8(+) and FOXP3(+) T Lymphocytes in Colorectal Cancer Provide Important Prognostic Clues. Br J Cancer (2014) 110:2551–9. doi:10.1038/bjc.2014.161
38. Ladoire, S, Martin, F, and Ghiringhelli, F. Prognostic Role of FOXP3+ Regulatory T Cells Infiltrating Human Carcinomas: The Paradox of Colorectal Cancer. Cancer Immunol Immunother (2011) 60(7):909–18. doi:10.1007/s00262-011-1046-y
39. Sharpe, AH, and Pauken, KE. The Diverse Functions of the PD1 Inhibitory Pathway. Nat Rev Immunol (2018) 18:153–67. doi:10.1038/nri.2017.108
40. Keir, ME, Butte, MJ, Freeman, GJ, and Sharpe, AH. PD-1 and its Ligands in Tolerance and Immunity. Annu Rev Immunol (2008) 26:677–704. doi:10.1146/annurev.immunol.26.021607.090331
41. Okazaki, T, and Honjo, T. The PD-1-PD-L Pathway in Immunological Tolerance. Trends Immunol (2006) 27:195–201. doi:10.1016/j.it.2006.02.001
42. Bardhan, K, Anagnostou, T, and Boussiotis, VA. The PD1:PD-L1/2 Pathway from Discovery to Clinical Implementation. Front Immunol (2016) 7:550. doi:10.3389/fimmu.2016.00550
43. Greenfield, EA, Nguyen, KA, and Kuchroo, VK. CD28/B7 Costimulation: A Review. Crit Rev Immunol (1998) 18(5):389–418. doi:10.1615/critrevimmunol.v18.i5.10
44. Calik, I, Calik, M, Turken, G, Ozercan, IH, Dagli, AF, Artas, G, et al. Intratumoral Cytotoxic T-Lymphocyte Density and PD-L1 Expression Are Prognostic Biomarkers for Patients with Colorectal Cancer. Medicina (2019) 55:723. doi:10.3390/medicina55110723
45. Berntsson, J, Eberhard, J, Nodin, B, Leandersson, K, Larsson, AH, Jirström, K, et al. Expression of Programmed Cell Death Protein 1 (PD-1) and its Ligand PD-L1 in Colorectal Cancer: Relationship with Sidedness and Prognosis. Oncoloimmunology (2018) 7:e1465165. doi:10.1080/2162402X.2018.1465165
46. Yi, M, Niu, M, Xu, L, Luo, S, and Wu, K. Regulation of PD-L1 Expression in the Tumor Microenvironment. J Hematol Oncol (2021) 14:10. doi:10.1186/s13045-020-01027-5
47. Akinleye, A, and Rasool, Z. Immune Checkpoint Inhibitors of PD-L1 as Cancer Therapeutics. J Hematol Oncol (2019) 12:92. doi:10.1186/s13045-019-0779-5
48. Noh, BJ, Kwak, JY, and Eom, DW. Immune Classification for the PD-L1 Expression and Tumour-Infiltrating Lymphocytes in Colorectal Adenocarcinoma. BMC Cancer (2020) 20:58. doi:10.1186/s12885-020-6553-9
49. Panda, A, Betigeri, A, Subramanian, K, Ross, JS, Pavlick, DC, Ali, S, et al. Identifying a Clinically Applicable Mutational Burden Threshold as a Potential Biomarker of Response to Immune Checkpoint Therapy in Solid Tumors. JCO Precis Oncol (2017) 2017:1–13. doi:10.1200/PO.17.00146
50. Liu, S, Gӧnen, M, Stadler, ZK, Weiser, MR, Hechtman, JF, Vakiani, E, et al. Cellular Localization of PD-L1 Expression in Mismatch-Repair-Deficient and Proficient Colorectal Carcinomas. Mod Pathol (2019) 32:110–21. doi:10.1038/s41379-018-0114-7
51. Li, Y, Liang, L, Dai, W, Cai, G, Xu, Y, Li, X, et al. Prognostic Impact of Programed Cell Death-1 (PD-1) and PD-Ligand 1 (PD-L1) Expression in Cancer Cells and Tumor Infiltrating Lymphocytes in Colorectal Cancer. Mol Cancer (2016) 15:55. doi:10.1186/s12943-016-0539-x
52. Ahtiainen, M, Wirta, EV, Kuopio, T, Seppälä, T, Rantala, J, Mecklin, JP, et al. Combined Prognostic Value of CD274 (PD-L1)/PDCDI (PD-1) Expression and Immune Cell Infiltration in Colorectal Cancer as Per Mismatch Repair Status. Mod Pathol (2019) 32:866–83. doi:10.1038/s41379-019-0219-7
53. Le, DT, Uram, JN, Wang, H, Bartlett, BR, Kemberling, H, Eyring, AD, et al. PD-1 Blockade in Tumors with Mismatch-Repair Deficiency. N Engl J Med (2015) 372:2509–20. doi:10.1056/NEJMoa1500596
54. Shen, Z, Gu, L, Mao, D, Chen, M, and Jin, R. Clinicopathological and Prognostic Significance of PD-L1 Expression in Colorectal Cancer: A Systematic Review and Meta-Analysis. World J Surg Oncol (2019) 17:4. doi:10.1186/s12957-018-1544-x
55. Buckowitz, A, Knaebel, HP, Benner, A, Bläker, H, Gebert, J, Kienle, P, et al. Microsatellite Instability in Colorectal Cancer Is Associated with Local Lymphocyte Infiltration and Low Frequency of Distant Metastases. Br J Cancer (2005) 92:1746–53. doi:10.1038/sj.bjc.6602534
56. Ganesh, K, Stadler, ZK, Cercek, A, Mendelsohn, RB, Shia, J, Segal, NH, et al. Immunotherapy in Colorectal Cancer: Rationale, Challenges and Potential. Nat Rev Gastroenterol Hepatol (2019) 16:361–75. doi:10.1038/s41575-019-0126-x
57. Yu, G, Wu, Y, Wang, W, Xu, J, Lv, X, Cao, X, et al. Low-dose Decitabine Enhances the Effect of PD-1 Blockade in Colorectal Cancer with Microsatellite Stability by Re-modulating the Tumor Microenvironment. Cell Mol Immunol (2019) 16(4):401–9. doi:10.1038/s41423-018-0026-y
58. Venderbosch, S, Nagtegaal, ID, Maughan, TS, Smith, CG, Cheadle, JP, Fisher, D, et al. Mismatch Repair Status and BRAF Mutation Status in Metastatic Colorectal Cancer Patients: A Pooled Analysis of the CAIRO, CAIRO2, COIN, and FOCUS Studies. Clin Cancer Res (2014) 20:5322–30. doi:10.1158/1078-0432.CCR-14-0332
60. Zarour, HM. Reversing T-Cell Dysfunction and Exhaustion in Cancer. Clin Cancer Res (2016) 22:1856–64. doi:10.1158/1078-0432.CCR-15-1849
61. Yassin, M, Sadowska, Z, Djurhuus, D, Nielsen, B, Tougaard, P, Olsen, J, et al. Upregulation of PD-1 Follows Tumour Development in the AOM/DSS Model of Inflammation-Induced Colorectal Cancer in Mice. Immunology (2019) 158:35–46. doi:10.1111/imm.13093
62. Ahn, J, Sinha, R, Pei, Z, Dominianni, C, Wu, J, Shi, J, et al. Human Gut Microbiome and Risk for Colorectal Cancer. J Natl Cancer Inst (2013) 105:1907–11. doi:10.1093/jnci/djt300
63. Huipeng, W, Lifeng, G, Chuang, G, Jiaying, Z, and Yuankun, C. The Differences in Colonic Mucosal Microbiota between normal Individual and colon Cancer Patients by Polymerase Chain Reaction-Denaturing Gradient Gel Electrophoresis. J Clin Gastroenterol (2014) 48:138–44. doi:10.1097/MCG.0b013e3182a26719
64. Kuwahara, T, Hazama, S, Suzuki, N, Yoshida, S, Tomochika, S, Nakagami, Y, et al. Intratumoural-infiltrating CD4 + and FOXP3 + T Cells as strong Positive Predictive Markers for the Prognosis of Resectable Colorectal Cancer. Br J Cancer (2019) 121:659–65. doi:10.1038/s41416-019-0559-6
65. Bourgeois, C, Veiga-Fernandes, H, Joret, AM, Rocha, B, and Tanchot, C. CD8 Lethargy in the Absence of CD4 Help. Eur J Immunol (2002) 32:2199–207. doi:10.1002/1521-4141(200208)32:8<2199:AID-IMMU2199>3.0.CO;2-L
66. Kurts, C, Carbone, FR, Barnden, M, Blanas, E, Allison, J, Heath, WR, et al. CD4+ T Cell Help Impairs CD8+ T Cell Deletion Induced by Cross-Presentation of Self-Antigens and Favors Autoimmunity. J Exp Med (1997) 186:2057–62. doi:10.1084/jem.186.12.2057
67. Xie, Y, Akpinarli, A, Maris, C, Hipkiss, EL, Lane, M, Kwon, EK, et al. Naive Tumor-specific CD4(+) T Cells Differentiated In Vivo Eradicate Established Melanoma. J Exp Med (2010) 207:651–67. doi:10.1084/jem.20091921
68. Gianchecchi, E, Delfino, DV, and Fierabracci, A. Recent Insights into the Role of the PD-1/PD-L1 Pathway in Immunological Tolerance and Autoimmunity. Autoimmun Rev (2013) 12:1091–100. doi:10.1016/j.autrev.2013.05.003
69. Kamphorst, AO, Wieland, A, Nasti, T, Yang, S, Zhang, R, Barber, DL, et al. Rescue of Exhausted CD8 T Cells by PD-1-Targeted Therapies is CD28-dependent. Science (2017) 355:1423–7. doi:10.1126/science.aaf0683
70. Gajewski, TF, Schreiber, H, and Fu, YX. Innate and Adaptive Immune Cells in the Tumor Microenvironment. Nat Immunol (2013) 14:1014–22. doi:10.1038/ni.2703
71. Palucka, AK, and Coussens, LM. The Basis of Oncoimmunology. Cell (2016) 164:1233–47. doi:10.1016/j.cell.2016.01.049
72. Sharma, P, and Allison, JP. The Future of Immune Checkpoint Therapy. Science (2015) 348:56–61. doi:10.1126/science.aaa8172
73. Reissfelder, C, Stamova, S, Gossmann, C, Braun, M, Bonertz, A, Walliczek, U, et al. Tumor-specific Cytotoxic T Lymphocyte Activity Determines Colorectal Cancer Patient Prognosis. J Clin Invest (2015) 125:739–51. doi:10.1172/JCI74894
74. Kwak, Y, Koh, J, Kim, DW, Kang, SB, Kim, WH, Lee, HS, et al. Immunoscore Encompassing CD3+ and CD8+ T Cell Densities in Distant Metastasis is a Robust Prognostic Marker for Advanced Colorectal Cancer. Oncotarget (2016) 7:81778–90. doi:10.18632/oncotarget.13207
75. Miller, TJ, Anyaegbu, CC, Lee-Pullen, TF, Spalding, LJ, Platell, CF, McCoy, MJ, et al. PD-L1+ Dendritic Cells in the Tumor Microenvironment Correlate with Good Prognosis and CD8+ T Cell Infiltration in colon Cancer. Cancer Sci (2021) 112:1173–83. doi:10.1111/cas.14781
76. Dahlin, AM, Henriksson, ML, Van Guelpen, B, Stenling, R, Oberg, A, Rutegård, J, et al. Colorectal Cancer Prognosis Depends on T-Cell Infiltration and Molecular Characteristics of the Tumor. Mod Pathol (2011) 24:671–82. doi:10.1038/modpathol.2010.234
77. Galon, J, Costes, A, Sanchez-Cabo, F, Kirilovsky, A, Mlecnik, B, Lagorce-Pagès, C, et al. Type, Density, and Location of Immune Cells within Human Colorectal Tumors Predict Clinical Outcome. Science (2006) 313:1960–4. doi:10.1126/science.1129139
78. Huang, CY, Chiang, SF, Ke, TW, Chen, TW, You, YS, Chen, WT, et al. Clinical Significance of Programmed Death 1 Ligand-1 (CD274/PD-L1) and Intra-tumoral CD8+ T-Cell Infiltration in Stage II-III Colorectal Cancer. Sci Rep (2018) 8:15658. doi:10.1038/s41598-018-33927-5
79. Anfossi, N, André, P, Guia, S, Falk, CS, Roetynck, S, Stewart, CA, et al. Human NK Cell Education by Inhibitory Receptors for MHC Class I. Immunity (2006) 25:331–42. doi:10.1016/j.immuni.2006.06.013
80. Coppola, A, Arriga, R, Lauro, D, Del Principe, MI, Buccisano, F, Maurillo, L, et al. NK Cell Inflammation in the Clinical Outcome of Colorectal Carcinoma. Front Med (Lausanne) (2015) 2:33. doi:10.3389/fmed.2015.00033
81. Schwartz, M, Zhang, Y, and Rosenblatt, JD. B Cell Regulation of the Anti-tumor Response and Role in Carcinogenesis. J Immunother Cancer (2016) 4:40. doi:10.1186/s40425-016-0145-x
82. Shimabukuro-Vornhagen, A, Schlößer, HA, Gryschok, L, Malcher, J, Wennhold, K, Garcia-Marquez, M, et al. Characterization of Tumor-Associated B-Cell Subsets in Patients with Colorectal Cancer. Oncotarget (2014) 5:4651–64. doi:10.18632/oncotarget.1701
83. Toor, SM, Sasidharan Nair, V, Murshed, K, Abu Nada, M, and Elkord, E. Tumor-Infiltrating Lymphoid Cells in Colorectal Cancer Patients with Varying Disease Stages and Microsatellite Instability-High/Stable Tumors. Vaccines (Basel) (2021) 9:64. doi:10.3390/vaccines9010064
84. Nirschl, CJ, and Drake, CG. Molecular Pathways: Coexpression of Immune Checkpoint Molecules: Signaling Pathways and Implications for Cancer Immunotherapy. Clin Cancer Res (2013) 19:4917–24. doi:10.1158/1078-0432.CCR-12-1972
85. Ren, D, Hua, Y, Yu, B, Ye, X, He, Z, Li, C, et al. Predictive Biomarkers and Mechanisms Underlying Resistance to PD1/PD-L1 Blockade Cancer Immunotherapy. Mol Cancer (2020) 19:19. doi:10.1186/s12943-020-1144-6
86. Turvey, SE, and Broide, DH. Innate Immunity. J Allergy Clin Immunol (2010) 125:S24–S32. doi:10.1016/j.jaci.2009.07.016
87. Chanmee, T, Ontong, P, Konno, K, and Itano, N. Tumor-associated Macrophages as Major Players in the Tumor Microenvironment. Cancers (Basel) (2014) 6(3):1670–90. doi:10.3390/cancers6031670
88. Sica, A, and Mantovani, A. Macrophage Plasticity and Polarization: In Vivo Veritas. J Clin Invest (2012) 122(3):787–95. doi:10.1172/JCI59643
89. Ngambenjawong, C, Gustafson, HH, and Pun, SH. Progress in Tumor-Associated Macrophage (TAM)-targeted Therapeutics. Adv Drug Deliv Rev (2017) 114:206–21. doi:10.1016/j.addr.2017.04.010
90. Liu, Y, and Cao, X. The Origin and Function of Tumor-Associated Macrophages. Cel Mol Immunol (2015) 12:1–4. doi:10.1038/cmi.2014.83
91. Qian, BZ, and Pollard, JW. Macrophage Diversity Enhances Tumor Progression and Metastasis. Cell (2010) 141:39–51. doi:10.1016/j.cell.2010.03.014
92. Lian, G, Chen, S, Ouyang, M, Li, F, Chen, L, Yang, J, et al. Colon Cancer Cell Secretes EGF to Promote M2 Polarization of TAM through EGFR/PI3K/AKT/mTOR Pathway. Technol Cancer Res Treat (2019) 18:1533033819849068. doi:10.1177/1533033819849068
93. Kobie, JJ, Wu, RS, Kurt, RA, Lou, S, Adelman, MK, Whitesell, LJ, et al. Transforming Growth Factor Inhibits the Antigen-Presenting Functions and Antitumor Activity of Dendritic Cell Vaccines. Cancer Res (2003) 63(8):1860–4.
94. Orsini, G, Legitimo, A, Failli, A, Ferrari, P, Nicolini, A, Spisni, R, et al. Defective Generation and Maturation of Dendritic Cells from Monocytes in Colorectal Cancer Patients during the Course of Disease. Int J Mol Sci (2013) 14(11):22022–41. doi:10.3390/ijms141122022
95. Hsu, YL, Chen, YJ, Chang, WA, Jian, SF, Fan, HL, Wang, JY, et al. Interaction between Tumor-Associated Dendritic Cells and colon Cancer Cells Contributes to Tumor Progression via CXCL1. Int J Mol Sci (2018) 19(8):2427. doi:10.3390/ijms19082427
96. Della Porta, M, Danova, M, Rigolin, GM, Brugnatelli, S, Rovati, B, Tronconi, C, et al. Dendritic Cells and Vascular Endothelial Growth Factor in Colorectal Cancer: Correlations with Clinicobiological Findings. Oncology (2005) 68(2-3):276–84. doi:10.1159/000086784
97. Ammendola, M, Sacco, R, Sammarco, G, Donato, G, Montemurro, S, Ruggieri, E, et al. Correlation between Serum Tryptase, Mast Cells Positive to Tryptase and Microvascular Density in colo-rectal Cancer Patients: Possible Biological-Clinical Significance. PLoS One (2014) 9(6):e99512. doi:10.1371/journal.pone.0099512
98. Suzuki, S, Ichikawa, Y, Nakagawa, K, Kumamoto, T, Mori, R, Matsuyama, R, et al. High Infiltration of Mast Cells Positive to Tryptase Predicts Worse Outcome Following Resection of Colorectal Liver Metastases. BMC Cancer (2015) 15:840. doi:10.1186/s12885-015-1863-z
99. Zhao, P, Zhou, P, Tang, T, Si, R, Ji, Y, Hu, X, et al. Levels of Circulating Mast Cell Progenitors and Tumour-Infiltrating Mast Cells in Patients with Colorectal Cancer. Oncol Rep (2022) 47:89. doi:10.3892/or.2022.8300
100. Sinha, P, Chornoguz, O, Clements, VK, Artemenko, KA, Zubarev, RA, Ostrand-Rosenberg, S, et al. Myeloid-derived Suppressor Cells Express the Death Receptor Fas and Apoptose in Response to T Cell-Expressed FasL. Blood (2011) 117(20):5381–90. doi:10.1182/blood-2010-11-321752
101. Fleming, V, Hu, X, Weber, R, Nagibin, V, Groth, C, Altevogt, P, et al. Targeting Myeloid-Derived Suppressor Cells to Bypass Tumor-Induced Immunosuppression. Front Immunol (2018) 9:398. doi:10.3389/fimmu.2018.00398
102. Dosset, M, Vargas, TR, Lagrange, A, Boidot, R, Végran, F, Roussey, A, et al. PD-1/PD-L1 Pathway: An Adaptive Immune Resistance Mechanism to Immunogenic Chemotherapy in Colorectal Cancer. Oncoimmunology (2018) 7(6):e1433981. doi:10.1080/2162402X.2018.1433981
103. McClellan, JL, Davis, JM, Steiner, JL, Enos, RT, Jung, SH, Carson, JA, et al. Linking Tumor-Associated Macrophages, Inflammation, and Intestinal Tumorigenesis: Role of MCP-1. Am J Physiol Gastrointest Liver Physiol (2012) 303(10):G1087–95. doi:10.1152/ajpgi.00252.2012
104. Vermeulen, L, De Sousa E Melo, F, van der Heijden, M, Cameron, K, de Jong, JH, Borovski, T, et al. Wnt Activity Defines colon Cancer Stem Cells and is Regulated by the Microenvironment. Nat Cel Biol (2010) 5:468–76. doi:10.1038/ncb2048
105. Hawinkels, LJ, Paauwe, M, Verspaget, HW, Wiercinska, E, van der Zon, JM, van der Ploeg, K, et al. Interaction with colon Cancer Cells Hyperactivates TGF-β Signaling in Cancer-Associated Fibroblasts. Oncogene (2014) 1:97–107. doi:10.1038/onc.2012.536
106. Vellinga, TT, den Uil, S, Rinkes, IH, Marvin, D, Ponsioen, B, Alvarez-Varela, A, et al. Collagen-rich Stroma in Aggressive colon Tumors Induces Mesenchymal Gene Expression and Tumor Cell Invasion. Oncogene (2016) 35:5263–71. doi:10.1038/onc.2016.60
107. Kalluri, R. The Biology and Function of Fibroblasts in Cancer. Nat Rev Cancer (2016) 9:582–98. doi:10.1038/nrc.2016.73
108. Mele, V, Muraro, MG, Calabrese, D, Pfaff, D, Amatruda, N, Amicarella, F, et al. Mesenchymal Stromal Cells Induce Epithelial-To-Mesenchymal Transition in Human Colorectal Cancer Cells through the Expression of Surface-Bound TGF-β. Int J Cancer (2014) 11:2583–94. doi:10.1002/ijc.28598
109. Brabletz, T, Kalluri, R, Nieto, MA, and Weinberg, RA. EMT in Cancer. Nat Rev Cancer (2018) 18:128–34. doi:10.1038/nrc.2017.118
110. Burger, GA, Danen, EHJ, and Beltman, JB. Deciphering Epithelial-Mesenchymal Transition Regulatory Networks in Cancer through Computational Approaches. Front Oncol (2017) 7:162. doi:10.3389/fonc.2017.00162
111. Jiang, Y, and Zhan, H. Communication between EMT and PD-L1 Signaling: New Insights into Tumor Immune Evasion. Cancer Lett (2020) 468:72–81. doi:10.1016/j.canlet.2019.10.013
112. Terry, S, Savagner, P, Ortiz-Cuaran, S, Mahjoubi, L, Saintigny, P, Thiery, JP, et al. New Insights into the Role of EMT in Tumor Immune Escape. Mol Oncol (2017) 11:824–46. doi:10.1002/1878-0261.12093
113. Zhan, HX, Zhou, B, Cheng, YG, Xu, JW, Wang, L, Zhang, GY, et al. Crosstalk between Stromal Cells and Cancer Cells in Pancreatic Cancer: New Insights into Stromal Biology. Cancer Lett (2017) 392:83–93. doi:10.1016/j.canlet.2017.01.041
114. Ma, HY, Liu, XZ, and Liang, CM. Inflammatory Microenvironment Contributes to Epithelial-Mesenchymal Transition in Gastric Cancer. World J Gastroenterol (2016) 22:6619–28. doi:10.3748/wjg.v22.i29.6619
115. Zippi, M, De Toma, G, Minervini, G, Cassieri, C, Pica, R, Colarusso, D, et al. Desmoplasia Influenced Recurrence of Disease and Mortality in Stage III Colorectal Cancer within Five Years after Surgery and Adjuvant Therapy. Saudi J Gastroenterol (2017) 23:39–44. doi:10.4103/1319-3767.199114
116. Ueno, H, Shinto, E, Shimazaki, H, Kajiwara, Y, Sueyama, T, Yamamoto, J, et al. Histologic Categorization of Desmoplastic Reaction: Its Relevance to the Colorectal Cancer Microenvironment and Prognosis. Ann Surg Oncol (2015) 22:1504–12. doi:10.1245/s10434-014-4149-9
117. Sueyama, T, Kajiwara, Y, Mochizuki, S, Shimazaki, H, Shinto, E, Hase, K, et al. Periostin as a Key Molecule Defining Desmoplastic Environment in Colorectal Cancer. Virchows Arch (2021) 478:865–74. doi:10.1007/s00428-020-02965-8
118. Becht, E, de Reyniès, A, Giraldo, NA, Pilati, C, Buttard, B, Lacroix, L, et al. Immune and Stromal Classification of Colorectal Cancer is Associated with Molecular Subtypes and Relevant for Precision Immunotherapy. Clin Cancer Res (2016) 22(16):4057–66. doi:10.1158/1078-0432.CCR-15-2879
119. Tougeron, D, Fauquembergue, E, Rouquette, A, Le Pessot, F, Sesboüé, R, Laurent, M, et al. Tumor-infiltrating Lymphocytes in Colorectal Cancers with Microsatellite Instability are Correlated with the Number and Spectrum of Frameshift Mutations. Mod Pathol (2009) 22(9):1186–95. doi:10.1038/modpathol.2009.80
120. Hodi, FS, O'Day, SJ, McDermott, DF, Weber, RW, Sosman, JA, Haanen, JB, et al. Improved Survival with Ipilimumab in Patients with Metastatic Melanoma. N Engl J Med (2010) 363(8):711–23. doi:10.1056/NEJMoa1003466
121. Topalian, SL, Hodi, FS, Brahmer, JR, Gettinger, SN, Smith, DC, McDermott, DF, et al. Safety, Activity, and Immune Correlates of Anti-PD-1 Antibody in Cancer. N Engl J Med (2012) 366(26):2443–54. doi:10.1056/NEJMoa1200690
122. Fan, J, Shang, D, Han, B, Song, J, Chen, H, Yang, JM, et al. Adoptive Cell Transfer: Is it a Promising Immunotherapy for Colorectal Cancer? Theranostics (2018) 8:5784–800. doi:10.7150/thno.29035
123. Beak, JH, and Kim, KJ. Expansion of Tumor-Infiltrating Lymphocytes and Their Potential for Application to Adoptive Cell Therapy in Patients with Colorectal Cancer. Eur J Surg Oncol (2019) 45(2):e58–9. doi:10.1016/j.ejso.2018.10.223
124. Koelzer, VH, Lugli, A, Dawson, H, Hädrich, M, Berger, MD, Borner, M, et al. CD8/CD45RO T-Cell Infiltration in Endoscopic Biopsies of Colorectal Cancer Predicts Nodal Metastasis and Survival. J Transl Med (2014) 12:81. doi:10.1186/1479-5876-12-81
125. Morris, M, Platell, C, and Iacopetta, B. Tumor-infiltrating Lymphocytes and Perforation in colon Cancer Predict Positive Response to 5-fluorouracil Chemotherapy. Clin Cancer Res (2008) 14(5):1413–7. doi:10.1158/1078-0432.CCR-07-1994
126. Halama, N, Michel, S, Kloor, M, Zoernig, I, Benner, A, Spille, A, et al. Localization and Density of Immune Cells in the Invasive Margin of Human Colorectal Cancer Liver Metastases are Prognostic for Response to Chemotherapy. Cancer Res (2011) 71(17):5670–7. doi:10.1158/0008-5472.CAN-11-0268
127. Valenzuela, G, Canepa, J, Simonetti, C, Solo de Zaldívar, L, Marcelain, K, González-Montero, J, et al. Consensus Molecular Subtypes of Colorectal Cancer in Clinical Practice: A Translational Approach. World J Clin Oncol (2021) 12(11):1000–8. doi:10.5306/wjco.v12.i11.1000
128. Eide, PW, Moosavi, SH, Eilertsen, IA, Brunsell, TH, Langerud, J, Berg, KCG, et al. Metastatic Heterogeneity of the Consensus Molecular Subtypes of Colorectal Cancer. NPJ Genom Med (2021) 6:59. doi:10.1038/s41525-021-00223-7
129. Fontana, E, Eason, K, Cervantes, A, Salazar, R, and Sadanandam, A. Context Matters-Consensus Molecular Subtypes of Colorectal Cancer as Biomarkers for Clinical Trials. Ann Oncol (2019) 30(4):520–7. doi:10.1093/annonc/mdz052
130. Kamal, Y, Schmit, SL, Hoehn, HJ, Amos, CI, and Frost, HR. Transcriptomic Differences between Primary Colorectal Adenocarcinomas and Distant Metastases Reveal Metastatic Colorectal Cancer Subtypes. Cancer Res (2019) 79:4227–41. doi:10.1158/0008-5472.CAN-18-3945
131. Akishima-Fukasawa, Y, Ino, Y, Nakanishi, Y, Miura, A, Moriya, Y, Kondo, T, et al. Significance of PGP9.5 Expression in Cancer-Associated Fibroblasts for Prognosis of Colorectal Carcinoma. Am J Clin Pathol (2010) 134:71–9. doi:10.1309/AJCPRJP39MIDSGBH
132. Glentis, A, Oertle, P, Mariani, P, Chikina, A, El Marjou, F, Attieh, Y, et al. Cancer-associated Fibroblasts Induce Metalloprotease-independent Cancer Cell Invasion of the Basement Membrane. Nat Commun (2017) 8:924. doi:10.1038/s41467-017-00985-8
133. Ren, J, Ding, L, Zhang, D, Shi, G, Xu, Q, Shen, S, et al. Carcinoma-associated Fibroblasts Promote the Stemness and Chemoresistance of Colorectal Cancer by Transferring Exosomal lncRNA H19. Theranostics (2018) 8(14):3932–48. doi:10.7150/thno.25541
134. Zhang, R, Qi, F, Shao, S, Li, G, and Feng, Y. Human Colorectal Cancer-Derived Carcinoma Associated Fibroblasts Promote CD44-Mediated Adhesion of Colorectal Cancer Cells to Endothelial Cells by Secretion of HGF. Cancer Cel Int (2019) 19:192. doi:10.1186/s12935-019-0914-y
135. Miyazaki, K, Togo, S, Okamoto, R, Idiris, A, Kumagai, H, Miyagi, Y, et al. Collective Cancer Cell Invasion in Contact with Fibroblasts through Integrin-α5β1/fibronectin Interaction in Collagen Matrix. Cancer Sci (2020) 111:4381–92. doi:10.1111/cas.14664
136. Unterleuthner, D, Neuhold, P, Schwarz, K, Janker, L, Neuditschko, B, Nivarthi, H, et al. Cancer-associated Fibroblast-Derived WNT2 Increases Tumor Angiogenesis in Colon Cancer. Angiogenesis (2020) 23:159–77. doi:10.1007/s10456-019-09688-8
137. Bauer, K, Michel, S, Reuschenbach, M, Nelius, N, von Knebel Doeberitz, M, Kloor, M, et al. Dendritic Cell and Macrophage Infiltration in Microsatellite-Unstable and Microsatellite -stable Colorectal Cancer. Fam Cancer (2011) 10:557–65. doi:10.1007/s10689-011-9449-7
138. Gulubova, MV, Ananiev, JR, Vlaykova, TI, Yovchev, Y, Tsoneva, V, Manolova, IM, et al. Role of Dendritic Cells in Progression and Clinical Outcome of colon Cancer. Int J Colorectal Dis (2012) 27:159–69. doi:10.1007/s00384-011-1334-1
139. Hu, Z, Ma, Y, Shang, Z, Hu, S, Liang, K, Liang, W, et al. Improving Immunotherapy for Colorectal Cancer Using Dendritic Cells Combined with Anti-programmed Death-Ligand In Vitro. Oncol Lett (2018) 15:5345–51. doi:10.3892/ol.2018.7978
140. Berntsson, J, Nodin, B, Eberhard, J, Micke, P, and Jirström, K. Prognostic Impact of Tumour-Infiltrating B Cells and Plasma Cells in Colorectal Cancer. Int J Cancer (2016) 139:1129–39. doi:10.1002/ijc.30138
141. Edin, S, Kaprio, T, Hagström, J, Larsson, P, Mustonen, H, Böckelman, C, et al. The Prognostic Importance of CD20+ B Lymphocytes in Colorectal Cancer and the Relation to Other Immune Cell Subsets. Sci Rep (2019) 9:19997. doi:10.1038/s41598-019-56441-8
142. Mullins, CS, Gock, M, Krohn, M, and Linnebacher, M. Human Colorectal Carcinoma Infiltrating B Lymphocytes are Active Secretors of the Immunoglobulin Isotypes A, G, and M. Cancers (Basel) (2019) 11:776. doi:10.3390/cancers11060776
143. Berntsson, J, Svensson, MC, Leandersson, K, Nodin, B, Micke, P, Larsson, AH, et al. The Clinical Impact of Tumour-Infiltrating Lymphocytes in Colorectal Cancer Differs by Anatomical Subsite: A Cohort Study. Int J Cancer (2017) 141:1654–66. doi:10.1002/ijc.30869
144. Digiacomo, N, Bolzacchini, E, Veronesi, G, Cerutti, R, Sahnane, N, Pinotti, G, et al. Neuroendocrine Differentiation, Microsatellite Instability, and Tumor-Infiltrating Lymphocytes in Advanced Colorectal Cancer with BRAF Mutation. Clin Colorectal Cancer (2019) 18(2):e251–60. doi:10.1016/j.clcc.2018.12.003
145. Glaire, MA, Domingo, E, Sveen, A, Bruun, J, Nesbakken, A, Nicholson, G, et al. Tumour-infiltrating CD8+ Lymphocytes and Colorectal Cancer Recurrence by Tumour and Nodal Stage. Br J Cancer (2019) 121:474–82. doi:10.1038/s41416-019-0540-4
146. Craig, SG, Humphries, MP, Alderdice, M, Bingham, V, Richman, SD, Loughrey, MB, et al. Immune Status Is Prognostic for Poor Survival in Colorectal Cancer Patients and is Associated with Tumour Hypoxia. Br J Cancer (2020) 123:1280–8. doi:10.1038/s41416-020-0985-5
147. Hartman, DJ, Frank, M, Seigh, L, Choudry, H, Pingpank, J, Holtzman, M, et al. Automated Quantitation of CD8-Positive T Cells Predicts Prognosis in Colonic Adenocarcinoma with Mucinous, Signet Ring Cell, or Medullary Differentiation Independent of Mismatch Repair Protein Status. Am J Surg Pathol (2020) 44:991–1001. doi:10.1097/PAS.0000000000001468
148. Fuchs, TL, Sioson, L, Sheen, A, Jafari-Nejad, K, Renaud, CJ, Andrici, J, et al. Assessment of Tumor-Infiltrating Lymphocytes Using International TILs Working Group (ITWG) System is a Strong Predictor of Overall Survival in Colorectal Carcinoma: A Study of 1034 Patients. Am J Surg Pathol (2020) 44:536–44. doi:10.1097/PAS.0000000000001409
149. Lalos, A, Tülek, A, Tosti, N, Mechera, R, Wilhelm, A, Soysal, S, et al. Prognostic Significance of CD8+ T-Cells Density in Stage III Colorectal Cancer Depends Od SDF-1 Expression. Sci Rep (2021) 11:775. doi:10.1038/s41598-020-80382-2
Keywords: colorectal cancer, tumor microenvironment, immune cells, tumorigenesis, consensus molecular subtypes, immunoscore
Citation: Wozniakova M, Skarda J and Raska M (2022) The Role of Tumor Microenvironment and Immune Response in Colorectal Cancer Development and Prognosis. Pathol. Oncol. Res. 28:1610502. doi: 10.3389/pore.2022.1610502
Received: 07 April 2022; Accepted: 28 June 2022;
Published: 21 July 2022.
Edited by:
Anna Sebestyén, Semmelweis University, HungaryCopyright © 2022 Wozniakova, Skarda and Raska. This is an open-access article distributed under the terms of the Creative Commons Attribution License (CC BY). The use, distribution or reproduction in other forums is permitted, provided the original author(s) and the copyright owner(s) are credited and that the original publication in this journal is cited, in accordance with accepted academic practice. No use, distribution or reproduction is permitted which does not comply with these terms.
*Correspondence: Maria Wozniakova, bWFyaWEud296bmlha292YUBmbm8uY3o=