- 1Institute of Pharmacy and Pharmacology, Hunan Provincial Key Laboratory of Tumor Microenvironment Responsive Drug Research, College of Basic Medical Science, Hengyang Medical School, University of South China, Hengyang, China
- 2Radiology Department, The First Affiliated Hospital of University of South China, Hengyang, China
- 3Changde Research Centre for Artificial Intelligence and Biomedicine, College of Life and Environmental Sciences, Hunan University of Arts and Science, Changde, China
To the Editor
One carbon (1C) unit metabolism plays a central role in supporting cell growth and proliferation in the body through the perception of cell glucose, amino acid and other nutritional statuses. The 1C metabolism pathway provides cellular components, including nucleotides, lipids, and proteins, for cell growth via folic acid and methionine cycles. The 1C metabolism pathway also generates glutathione and S-adenosylmethionine to maintain cellular redox status and epigenetic status with cytosolic or mitochondrial folate metabolism. Targeting 1C unit metabolic enzymes and downstream nucleic acid metabolic enzymes is seen as a viable cancer treatment method since the metabolism of amino acids (such as serine and glycine) and the 1C units they provide are sufficient to promote tumor growth [1].
Mitochondrial 1C flux is consistently overexpressed in cancer and supplied by the folic acid cycle and the mitochondrial serine-hydroxymethyltransferase (SHMT2), while cytosolic 1C flux is induced by the cytosolic enzyme SHMT1. Mitochondrial and cytosolic 1C flux is responsible for DNA replication and methylation. However, several studies have indicated that blocking mitochondrial 1C flux with SHMT2 inhibitors cannot abolish the neoplasia of hepatocellular cancer cells absolutely, whereas targeting mitochondrial and cytosolic 1C flux with dual SHMT1/2 inhibitors can restrain the formation of transplanted tumors completely. Hence, it is hinted that cytosolic 1C flux may also play an unexpected role in tumor progression under some nutrient availability.
Lee et al. [2] identified that tumor reliance on cytosolic versus mitochondrial 1C flux depends on folate levels, thereby challenging the current opinion that mitochondrial 1C flux is the sole contributor of 1C units in tumors. To demonstrate that 1C flux is determined by folic acid levels in cancer cells, the author analyzed cytosolic versus mitochondrial 1C metabolic flux in various tumors under normal physiological folate concentration. The results showed that SHMT1-mediated cytosolic 1C metabolic flux is the primary source of 1C units in a series of cancers, including T cell acute lymphoblastic leukemia, glioblastoma, and non-small-cell lung carcinoma under normal physiological folate concentration, whereas mitochondrial 1C flux is overly repressed, suggesting that tumor-specific reliance on cytosolic 1C flux depends on poor folate availability. In this study, the 1C metabolic flux of cancer cells is switched primarily toward the cytosolic folate cycle through SHMT1 to face nutrient availability under normal physiological folate concentration. Furthermore, the increased reliance on SHMT1 for producing 1C units is followed by a decrease in whole-cell SHMT flux, indicating a prominent drop specifically in SHMT2 flux under normal physiological folate concentration. It has been identified that cytoplasmic SHMT1 is overexpressed in lung cancer. SHMT1-dependent apoptosis is due to an increased accumulation of uracil during DNA replication [3]. In proliferating cancer cells, cytosolic 1C pathway flux through SHMT1 is essential to compensate for DNA replication and maintain tumor growth under nutrient-poor conditions. Notably, metabolic switch generally exists in tumor cells under poor environmental conditions. Cancer cells can switch their metabolic phenotypes to adapt to changes in their surroundings [4]. For example, brain tumor cells accommodate deprivation and preserve their survival by upregulating glucose transporters and switching to a more glycolytic phenotype. The metabolic switch helps breast cancer cells survive under hypoxia. Human high-grade glioma cancer cells also undergo metabolic shifts in response to the chemotherapy environment and induce therapy resistance. More importantly, metabolic switch inhibition prominently alleviates tumor progression, suggesting that targeting metabolic switch can be a promising strategy for tumor treatment [2].
Mechanically, 1C metabolic switch toward tumor-specific cytosolic 1C flux is mainly attributed to the decreased expression of SLC19A1 that encodes the reduced folate carrier (RFC). Low RFC expression causes the poor capacity to retain intracellular folates in cancer cells, thereby resulting in SHMT1-mediated reliance on cytosolic 1C metabolic flux. Furthermore, silencing SHMT1 with low SLC19A1 expression inhibits tumor growth by impairing pyrimidine biosynthesis in vitro and in vivo, whereas silencing SHMT1 with SLC19A1 overexpression cannot alleviate tumor growth. Consequently, a decrease in SLC19A1 makes tumors susceptible to SHMT1 and determines the 1C metabolic switch toward cytosolic 1C metabolic flux under physiological folate conditions. Inhibiting SHMT1 offers potential therapeutic options for tumor patients with low SLC19A1 expression.
In the future, SLC19A1 may be a marker for tumor patients that have an increased reliance on cytosolic 1C metabolic flux. Inhibiting SHMT1 expression may be an emerging strategy for tumor patients with low SLC19A1 expression (Figure 1). Currently, most drugs that selectively target SHMT2, such as antifolates lometrexol and pemetrexed, are recognized as anti-cancer drugs with long-term activity. However, the potential function of SHMT1 inhibitors has always been concerning for treating tumor diseases (Table 1). For example, compound 2.12, a derivative of the pyrazolopyran scaffold, has a lower dissociation constant when binding to the SHMT1 enzyme-serine complex and is preferred to inhibit SHMT1, eventually leading to cell death in lung cancer cells [5]. Mimosine, a naturally occurring plant amino acid, can chelate zinc, thereby inhibiting the SHMT1 promoter and blocking the its transcription [6]. Arsenic trioxide can increase the ubiquitin degradation of methylenetetrahydrofolate dehydrogenase 1 (MTHFD1) and SHMT1 to inhibit nuclear de novo thymidylate (dTMP) biosynthesis, thus increasing genome instability [7]. The spiro-dihydroindene analogs with a spirocyclic scaffold potently inhibit human cytosolic and plasmodial SHMT1 [8]. Tetrahydrofolate (THF) decreases SHMT1 production through the pH dependent-substrate inhibition [9]. 3-bromopyruvate (3BP) can bind to the active site of SHMT1 (Cys204) to form the enzyme-3BP complex, which completely inhibits human SHMT1 to achieve an anti-tumor effect [10]. The SHMT1-targeted inhibitor AGF347, a novel pyrrolo [3,2-d] pyrimidine compound, shows anti-tumor efficacy in HCT116 colon cancer, H460 non-small cell lung cancer (NSCLC), and MIA PaCa-2 pancreatic cancer [11]. However, AGF347 is not selective for SHMT1 and SHMT2. Besides, antifolate compounds are used clinically for treating cancers by inhibiting SHMT1. Lometrexol is an SHMT2 inhibitor and one of the best SHMT1 inhibitors in a panel of antifolate drugs [12]. MicroRNA-198 can downregulate SHMT1 expression to suppress the proliferation of lung adenocarcinoma cells, which shows a more effective alteration than silencing SHMT1 with siRNA [13]. Notably, microRNA-218-5p inhibits SHMT1 to suppress the natural killer (NK) cells and exerts a negative effect on lung adenocarcinoma [14].
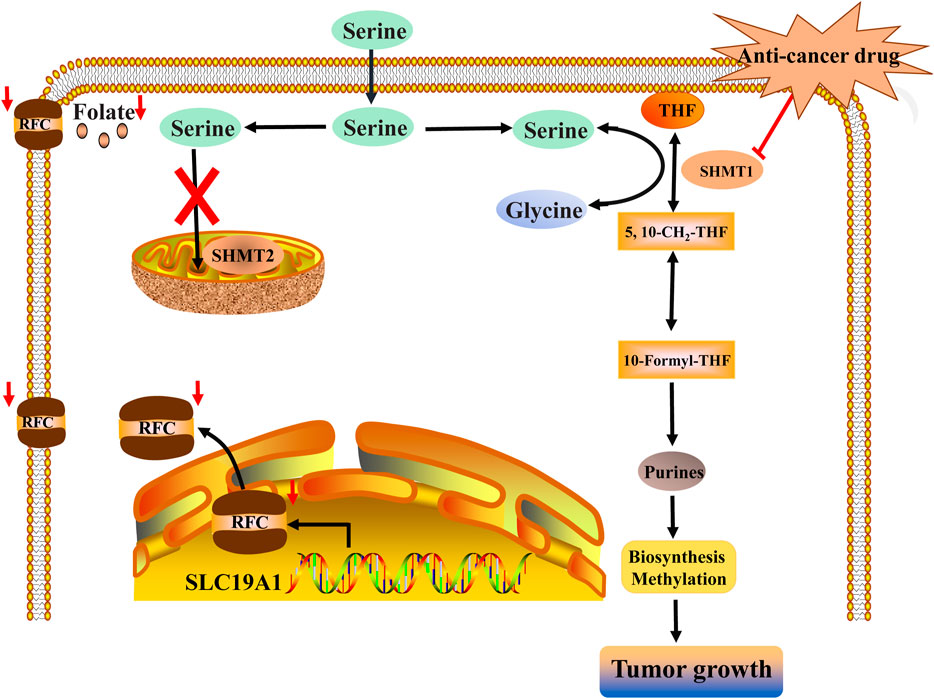
FIGURE 1. Inhibiting SHMT1 may be a vital target for a series of tumor diseases with low SLC19A1 expression. Low expression of SLC19A1 causes the poor capacity of cancer cells to retain intracellular folates, thereby resulting in a low level of folate conditions. Then, a 1C metabolic switch toward cytosolic 1C flux rather than mitochondrial 1C flux enables cancer cells to depend on SHMT1. Finally, SHMT-mediated cytosolic 1C metabolic flux can provide DNA replication and methylation for the growth of tumors with low SLC19A1 expression. Inhibiting SHMT1 can impair the growth of tumors with low SLC19A1 expression.
This paper reveals the diversity of 1C metabolism under different conditions in cancer cells. To better accommodate the poor environment, cancer cells are forced to adopt metabolic switch to maintain their growth and development. Selective inhibition of the specific isoform of SHMT may be a key target for many human tumors with metabolic switch. Nevertheless, the relationship between SHMT1 and SHMT2 in human tumors is extremely complicated. It has been demonstrated that SHMT1 can utilize its RNA-binding function to bind the 5′untranslated region of the SHMT2 transcript (UTR2) and control the function of SHMT2-expressed cancer cells. Inversely, the transfection of UTR2 in cancer cells can also modulate SHMT1 activity and reduce cell viability, suggesting that it is of great necessity for us to give more studies on the relationship between SHMT1 and SHMT2. Going forward, it needs to be carefully evaluated how to better utilize 1C metabolism to target tumors.
Author Contributions
Conception and design: LC and HH; manuscript writing: ZC; provision of instruction and expert advice: HZ; final approval of manuscript: all authors.
Funding
This study was supported by the National Natural Science Foundation of China (81973326), the Scientific Research Fund Project of Hunan Provincial Health Commission (20201901), the Natural Science Foundation of Hunan Province (2019JJ50523 and 2019JJ40255). The Scientific Research Youth Foundation of Hu’nan Provincial Education Department (21B0610).
Conflict of Interest
The authors declare that the research was conducted in the absence of any commercial or financial relationships that could be construed as a potential conflict of interest.
Acknowledgments
We are profoundly grateful to my tutor, Prof. Chen whose illuminating instruction and expert advice have guided us for my writing of this thesis.
Abbreviations
1C units, One-carbon units; DMEM, Dulbecco’s modified eagle medium; FBP1, Fructose-1,6-biphosphatase; MTHFD1, Methylenetetrahydrofolate dehydrogenase 1; RFC, The reduced folate carrier; RPMI, Roswell Park Memorial Institute; SHMT1, Cytosolic serine-hydroxymethyltransferase; SHMT2, Mitochondrial serine-hydroxymethyltransferase; TSH, Tetrahydrofolate; UTR2, the 5′untranslated region of the SHMT2 transcript.
References
1. Ducker, GS, Chen, L, Morscher, RJ, Ghergurovich, JM, Esposito, M, Teng, X, et al. Reversal of Cytosolic One-Carbon Flux Compensates for Loss of the Mitochondrial Folate Pathway. Cell Metab (2016) 24(4):640–1. doi:10.1016/j.cmet.2016.09.011
2. Lee, WD, Pirona, AC, Sarvin, B, Stern, A, Nevo-Dinur, K, Besser, E, et al. Tumor Reliance on Cytosolic versus Mitochondrial One-Carbon Flux Depends on Folate Availability. Cell Metab (2020) 33:190–8. doi:10.1016/j.cmet.2020.12.002
3. Paone, A, Marani, M, Fiascarelli, A, Rinaldo, S, Giardina, G, Contestabile, R, et al. SHMT1 Knockdown Induces Apoptosis in Lung Cancer Cells by Causing Uracil Misincorporation. Cell Death Dis (2014) 5:e1525. doi:10.1038/cddis.2014.482
4. Vlashi, E, Lagadec, C, Vergnes, L, Matsutani, T, Masui, K, Poulou, M, et al. Metabolic State of Glioma Stem Cells and Nontumorigenic Cells. Proc Natl Acad Sci U.S.A (2011) 108(38):16062–7. doi:10.1073/pnas.1106704108
5. Marani, M, Paone, A, Fiascarelli, A, Macone, A, Gargano, M, Rinaldo, S, et al. A Pyrazolopyran Derivative Preferentially Inhibits the Activity of Human Cytosolic Serine Hydroxymethyltransferase and Induces Cell Death in Lung Cancer Cells. Oncotarget (2016) 7(4):4570–83. doi:10.18632/oncotarget.6726
6. Perry, C, Sastry, R, Nasrallah, IM, and Stover, PJ. Mimosine Attenuates Serine Hydroxymethyltransferase Transcription by Chelating Zinc. J Biol Chem (2005) 280(1):396–400. doi:10.1074/jbc.m410467200
7. Kamynina, E, Lachenauer, ER, DiRisio, AC, Liebenthal, RP, Field, MS, and Stover, PJ. Arsenic Trioxide Targets MTHFD1 and SUMO-dependent Nuclear De Novo Thymidylate Biosynthesis. Proc Natl Acad Sci U S A (2017) 114(12):E2319–E2326. doi:10.1073/pnas.1619745114
8. Schwertz, G, Witschel, MC, Rottmann, M, Leartsakulpanich, U, Chitnumsub, P, Jaruwat, A, et al. Potent Inhibitors ofPlasmodialSerine Hydroxymethyltransferase (SHMT) Featuring a Spirocyclic Scaffold. ChemMedChem (2018) 13(9):931–43. doi:10.1002/cmdc.201800053
9. Tramonti, A, Nardella, C, di Salvo, ML, Barile, A, Cutruzzolà, F, and Contestabile, R. Human Cytosolic and Mitochondrial Serine Hydroxymethyltransferase Isoforms in Comparison: Full Kinetic Characterization and Substrate Inhibition Properties. Biochemistry (2018) 57(51):6984–96. doi:10.1021/acs.biochem.8b01074
10. Paiardini, A, TramontiSchirch, A, Schirch, D, Guiducci, G, di Salvo, ML, Fiascarelli, A, et al. Differential 3-bromopyruvate Inhibition of Cytosolic and Mitochondrial Human Serine Hydroxymethyltransferase Isoforms, Key Enzymes in Cancer Metabolic Reprogramming. Biochim Biophys Acta (Bba) - Proteins Proteomics (2016) 1864(11):1506–17. doi:10.1016/j.bbapap.2016.08.010
11. Dekhne, AS, Shah, K, Ducker, GS, Katinas, JM, Wong-Roushar, J, Nayeen, MJ, et al. Novel Pyrrolo[3,2-D]pyrimidine Compounds Target Mitochondrial and Cytosolic One-Carbon Metabolism with Broad-Spectrum Antitumor Efficacy. Mol Cancer Ther (2019) 18(10):1787–99. doi:10.1158/1535-7163.mct-19-0037
12. Scaletti, E, Jemth, AS, Helleday, T, and Stenmark, P. Structural Basis of Inhibition of the Human Serine Hydroxymethyltransferase SHMT 2 by Antifolate Drugs. FEBS Lett (2019) 593(14):1863–73. doi:10.1002/1873-3468.13455
13. Wu, S, Zhang, G, Li, P, Chen, S, Zhang, F, Li, J, et al. miR-198 Targets SHMT1 to Inhibit Cell Proliferation and Enhance Cell Apoptosis in Lung Adenocarcinoma. Tumor Biol (2016) 37(4):5193–202. doi:10.1007/s13277-015-4369-z
Keywords: One-carbon units, SHMT1, SHMT2, RPMI, TSH, MTHFD1
Citation: Chen Z, Zhou H, Hu H and Chen L (2022) Blocking the Metabolic Switch Toward Cytosolic 1C Flux: A Novel Therapeutic Approach for Tumors With Low SLC19A1 Expression. Pathol. Oncol. Res. 28:1610337. doi: 10.3389/pore.2022.1610337
Received: 28 January 2022; Accepted: 30 March 2022;
Published: 22 April 2022.
Edited by:
Anna Sebestyén, Semmelweis University, HungaryCopyright © 2022 Chen, Zhou, Hu and Chen. This is an open-access article distributed under the terms of the Creative Commons Attribution License (CC BY). The use, distribution or reproduction in other forums is permitted, provided the original author(s) and the copyright owner(s) are credited and that the original publication in this journal is cited, in accordance with accepted academic practice. No use, distribution or reproduction is permitted which does not comply with these terms.
*Correspondence: Haoliang Hu, aGFvbGlhbmdodTZAMTI2LmNvbQ==; Linxi Chen, bHhjaGVuNkAxMjYuY29t
†These authors have contributed equally to this work